40 Changes in Enzyme Activity
It would seem ideal to have a scenario in which all of an organism’s enzymes existed in abundant supply and functioned optimally under all cellular conditions, in all cells, at all times. However, there are several reasons that this does not happen. Cellular needs and conditions constantly vary from cell to cell, and change within individual cells over time. The required enzymes of stomach cells differ from those of fat storage cells, skin cells, blood cells, and nerve cells. Furthermore, a digestive organ cell works much harder to process and break down nutrients during the time that closely follows a meal compared with many hours after a meal. As these cellular demands and conditions vary, so must the amounts and functionality of different enzymes.
Since the rates of biochemical reactions are controlled by activation energy, and enzymes lower and determine activation energies for chemical reactions, the relative amounts and functioning of the variety of enzymes within a cell ultimately determine which reactions will proceed and at what rates. This determination is tightly controlled in cells.
Regulation
Enzymes can be regulated in ways that either promote or reduce enzyme activity. There are many kinds of molecules that inhibit or promote enzyme function, and various mechanisms by which they do so.
- In some cases of enzyme inhibition, an inhibitor molecule is similar enough to a substrate that it can bind to the active site and simply block the substrate from binding.
- In other cases, an inhibitor molecule binds to the enzyme in a location other than the active site, called an allosteric site, but still manages to block substrate binding to the active site.
- There are activators as well as inhibitors. Activators bind to locations on an enzyme away from the active site, inducing a conformational change that increases the affinity of the enzyme’s active site(s) for its substrate(s) (Figure 1).
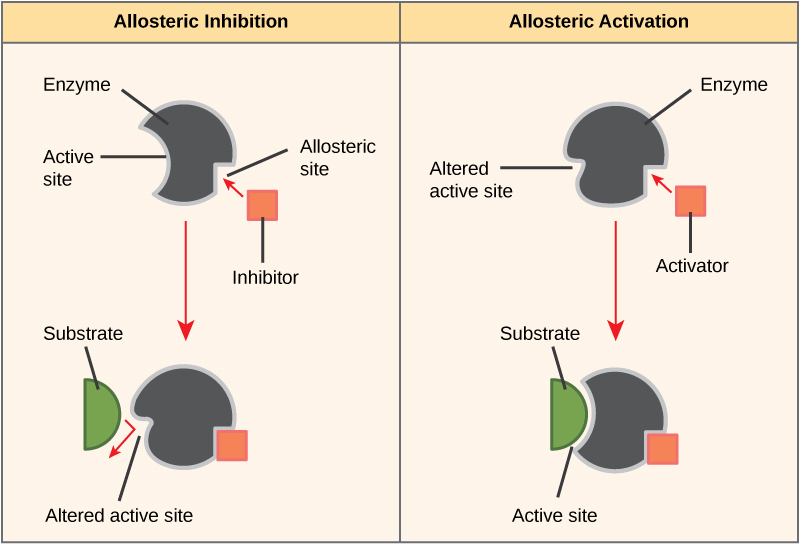
Cofactors and Coenzymes
Many enzymes do not work optimally, or even at all, unless bound to other specific non-protein helper molecules. They may bond either temporarily through ionic or hydrogen bonds, or permanently through stronger covalent bonds. Binding to these molecules promotes optimal shape and function of their respective enzymes. Two examples of these types of helper molecules are cofactors and coenzymes. Cofactors are inorganic ions such as ions of iron and magnesium. Coenzymes are organic helper molecules, those with a basic atomic structure made up of carbon and hydrogen. Like enzymes, these molecules participate in reactions without being changed themselves and are ultimately recycled and reused. Vitamins are the source of coenzymes. Some vitamins are the precursors of coenzymes and others act directly as coenzymes. Vitamin C is a direct coenzyme for multiple enzymes that take part in building the important connective tissue, collagen. Therefore, enzyme function is, in part, regulated by the abundance of various cofactors and coenzymes, which may be supplied by an organism’s diet or, in some cases, produced by the organism.
Denaturing
Increasing the environmental temperature generally increases reaction rates, enzyme-catalyzed or otherwise. However, temperatures outside of an optimal range reduce the rate at which an enzyme catalyzes a reaction. Hot temperatures will eventually cause enzymes to denature, an irreversible change in the three-dimensional shape and therefore the function of the enzyme (Figure 2). Enzymes are also suited to function best within a certain pH and salt concentration range, and, as with temperature, extreme pH, and salt concentrations can cause enzymes to denature.
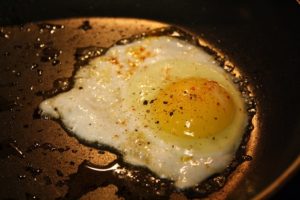
For example, the enzyme amylase is found in saliva, where it functions to break down starch (a polysaccharide – carbohydrate chain) into smaller sugars. Note that in this example, amylase is the enzyme, starch is the substrate, and smaller sugars are the product. The pH of saliva is typically between 6.2 and 7.6, with roughly 6.7 being the average. The optimum pH of amylase is between 6.7 and 7.0, which is close to neutral (Figure 3). The optimum temperature for amylase is close to 37ºC (which is human body temperature).

References
Unless otherwise noted, images on this page are licensed under CC-BY 4.0 by OpenStax.
Text adapted from: OpenStax, Concepts of Biology. OpenStax CNX. May 18, 2016 http://cnx.org/contents/b3c1e1d2-839c-42b0-a314-e119a8aafbdd@9.10