1.3 The Environment is a System
Ok, so the environment is everything, all of the biotic (living) and abiotic (non-living) parts of nature, of our world. The trees, the grass, the beetles, the bacteria, the water, the soil, the wind, even us humans… but is it just a jumbled collection of things? No, all those things are profoundly, intimately, and dynamically interconnected to each other, in ways which may be obvious or more subtle. If you change one part, the impacts of that change can ripple out throughout the system as a whole, in sometimes unexpected ways.
But a lot of what science does is try to predict, to help us know what to expect. So in order to study the environment in all it’s details and complexity, it’s helpful to start by thinking about what systems are, and learn something about how we study them.
Introduction to Systems and Systems Thinking
In an increasingly complex and interconnected world, thinking systemically can help us to understand, communicate, and address challenges we face. Yet if you want to start using this approach, it can be difficult to know how to start. Systems thinking is both an approach to seeing the world in a way that makes connections and relationships more visible and improves our decision-making abilities, and a set of methods and tools. So we’ll spend some time here on an overview of what systems are and ways to think about them. We’ll use systems thinking and systems thinking tools throughout the term, and you may find them useful in problem solving, and in understanding many aspects of life and the world you live in.
What is a System?
In order to think in systems, we must first understand what this term means. We encounter systems in many different contexts and situations—from circulatory systems to climate systems to healthcare systems to transportation systems. But what is a system? How would we know a system if we saw one, and why is it important to understand systems at all?
A system is a group of two or more related parts (sometimes referred to as elements, components, or stocks) that interact over time to form a whole that has a purpose, function, or behavior. We can conceive of systems as physical entities that we can observe and empirically examine (like a tree or a subway system), or as abstract constructs we can use to understand our world (like a model of a cell or of the solar system). A system includes both individual parts, and also the relationships that hold the parts together—these can be physical flows (for example, the neural signals that allow us to sense our environment), or simply flows of information in a social system. Many parts can form a whole, but unless they depend on and interact with each other, they are simply a collection. Consider a jar of dried basil, a jar of cinnamon, and a jar of paprika—a group of spices that comprise a spice rack. Their function does not change if you add or remove spice jars or re-arrange them, because the spice rack is simply a collection. In contrast, your body is a system composed of multiple different, interacting, and interdependent organs or nested subsystems. A set of parts, arranged and connected, is essential for you to survive. Furthermore, these components can function together in ways that would not be possible for each part on its own, and as a system, exhibit properties that emerge only when parts interact with a wider whole (also known as emergent properties) (Box 1).
Box 1. Handy question guide: is it a system, or just a collection of stuff? (adapted from Meadows 2008).
- Are the parts identifiable? If yes, then…
- Do the parts interact with each other? If yes, then…
- Is there a difference between the combined interactions of the parts (or function of the parts together) compared to how each part behaves on its own? If yes, then…
- Does the function persist in a variety of situations?
If yes, then… it’s a system!
Investigating the parts and interrelationships of systems helps us understand how they function (a term usually used for nonhuman systems, like a computer) or their purpose (a term usually used for human systems, like a government). Often the best way to discern the function (e.g., delivering potable water from a faucet) or purpose (e.g., educating a student) of a system is to observe how the system operates over time, in terms of growth, decline, oscillation, stasis, or evolution.
All systems have boundaries that define what is “in” and “out” of the system. And most bounded systems are nested or hierarchical; there can be purposes within purposes. Hierarchy is the arrangement of subsystems organized into larger and larger systems. An example of a hierarchy is an individual cell within your heart, which, in turn, is part of your circulatory system, which is in turn a subsystem of your body. A hierarchy not only gives a system stability and resilience but also reduces the amount of information that any part of the system has to track. For example, a ship’s captain might not need to know about the individual action of every individual crew member because they trust those leaders to make appropriate decisions at their level.
Sorting Systems
Systems are often sorted into three general categories: simple, complicated, and complex (a fourth category, chaotic, is used to describe a system with weak connections between parts and no discernible patterns in function) (Figure 1). It is important to understand the differences between these systems because they each call for different approaches for stakeholders who are managing the system or working to move it towards producing more desired outcomes.
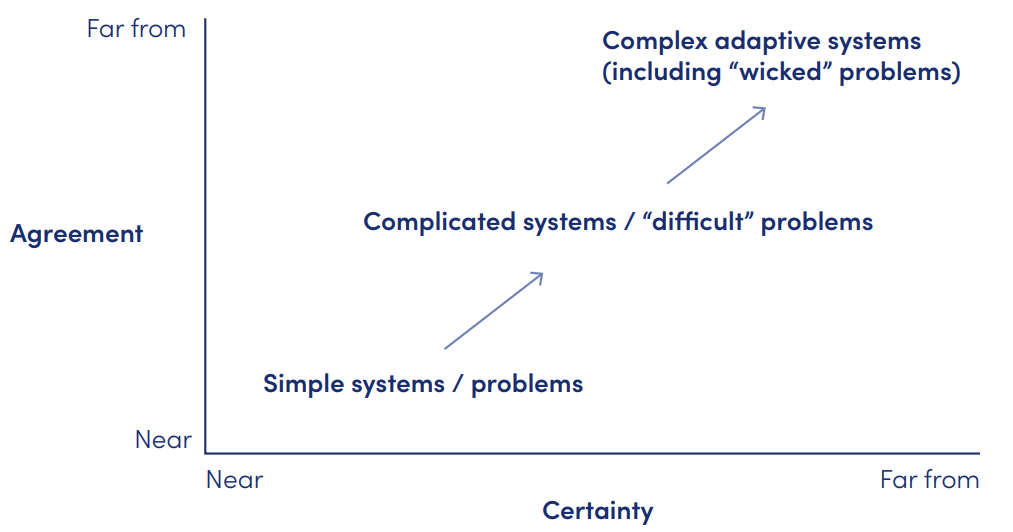
- Simple systems are easily knowable—they have few parts with stable relationships, and the function of the system is predictable, for example, a pet goldfish in a bowl represents a simple system. The process of managing a simple system, or caring for your goldfish so it can survive and thrive, is relatively straightforward and does not deviate significantly given different contexts.
- Complicated systems are not simple but are definable, and subsequently solvable, and may require high levels of expertise in specialized fields to maintain the system. Systems can be technically complicated or socially complicated, or some combination of both. They do not adapt but rather have a high degree of certainty of outcome; for example, a computer represents a technically complicated system where outcomes can be determined: the computer is working properly, or it’s not. Indicators of success or progress in managing complicated systems are directly linked through cause and effect. For the computer example, successful management requires a team with the proper expertise to maintain the system, diagnose malfunctions, and make repairs. A socially complicated system may involve different stakeholders with different perspectives and values; for example, scheduling times for classroom use in schools may be socially complicated, but it is
generally solvable in the sense that a classroom schedule can be agreed upon and implemented. For socially complicated issues, the key to moving forward often comes down to building relationships, fnding common ground, and creating space for respectful dialogue and differences of opinion. - Most environmental systems are even more complex. In contrast to simple and complicated systems, complex adaptive systems (CAS) have many different parts, and non-linear relationships with feedback loops across time and space (non-linear means that cause and effect of a system are not in proportion to one another, sometimes referred to as tipping points). While some complex adaptive systems are entirely human, these systems are usually a mix of interrelated human and non-human subsystems, and are dynamic, unpredictable, and change over time. They can also self-organize, evolve, and adapt.
- Self-organization is the ability of systems to structure themselves without central control, to create new parts or relationships, and to learn and evolve in complexity.
- Self-organized systems can exhibit emergent properties, a phenomenon that can refer to any kind of learning or new pattern that emerges from the complex interactions of a system’s parts (the elegant changing shape of a flock of flying birds is an example of emergence).
Image credit: xkcd.com
The human immune system is an exquisite example of a complex adaptive system that is able to learn to defend the body against unknown pathogens through self-organizing, adaptive immunity. Democracy is another example of a complex adaptive system, continually evolving in unpredictable ways in numerous social and political contexts through time and space. If we want to influence a complex adaptive system to improve its trends and enable desired outcomes, one-size-fits-all approaches are unlikely to yield success. When we try to predict, specify, design, and force a CAS in a certain way, we may be misguidedly treating it as just a complicated system. But if it is complex, different approaches are likely required to understand it and manage it. The approaches we use to understand and maintain a complicated system like a computer will not yield success when applied to a CAS like the internet.
Management of complex adaptive systems is especially tricky for so-called “wicked” or intractable problems that are unsolvable in the conventional sense. Problems are termed “wicked” when
- they have multiple, often undefined, causes
- little to no agreement on how to improve a situation
- requires collective action among stakeholders with differing values and contested understandings of the problem
- cause-and-effect relationships that only became clear after the effects have already emerged.
Human disease, refugee migration, and climate change are examples of wicked problems. While wicked problems have long, uncertain timescales and will never be “solved” in the conventional sense, we can work to understand these particular systems and make decisions that alter their function in ways that can promote progress towards desired outcomes.
What is Systems Thinking?
Investigating systems through “systems thinking” opens up countless possibilities for understanding and influencing the world around us. While there is no widely accepted definition for the term. Their proposed definition is that systems thinking is “a set of synergistic analytic skills used to improve the capability of identifying and understanding systems, predicting their behaviors, and devising modifications to them in order to produce desired effects.” Systems thinking is both
- an approach to seeing the world—in a way that makes connections and relationships more visible and improves our decisionmaking abilities—and
- a set of methods and tools
When we use systems thinking as a way of seeing (a systems “lens”) we see that the world is made up of parts that, in turn, connect and interact in dynamic ways to form a whole, whether we are looking at food systems, economies, or ourselves. With this ability to “see” where in a system and the relationships within it problems seem to originate, system thinkers can then think about ways to change the system, communicate with others to create new ways of thinking and seeing, and through this shared understanding, plan more effectively for the future. The key for systems thinking is that it is NOT about prediction or control, but about raising more questions to enhance understanding and seeking opportunities to “nudge” system function in desired directions (Box 2). Systems thinking helps with seeing that there is frequently not one single solution to a problem, but a set of coordinated actions that guide the system towards a desired state or outcome.
Box 2. Questions a systems thinker asks when faced with a complex issue
- What happened?
- What recurring events are we noticing?
- What length of time is long enough to see patterns in system function?
- What structures may be determining the function we see?
- What underlying beliefs, values, or assumptions are at play, including from or within ourselves?
- What are the feedbacks in this system?
- How can we defne a boundary around this system? What is appropriate and useful for our goals?
- What other perspectives, methodologies, or disciplines might help us more fully understand this issue?
- Where in this system could we make small shifts that would make a big difference? What trade-offs exist? Is there a potential for unintended consequences?
Each of these questions relate to how a system is structured and how it operates over time. Systems thinking emphasizes relationships and interactions between parts of a system, interrogating the nature of the relationships and discerning patterns or trends emerging from these relationships. System thinkers seek root causes, or the connections between events and the underlying structure of the system (Figure 2).
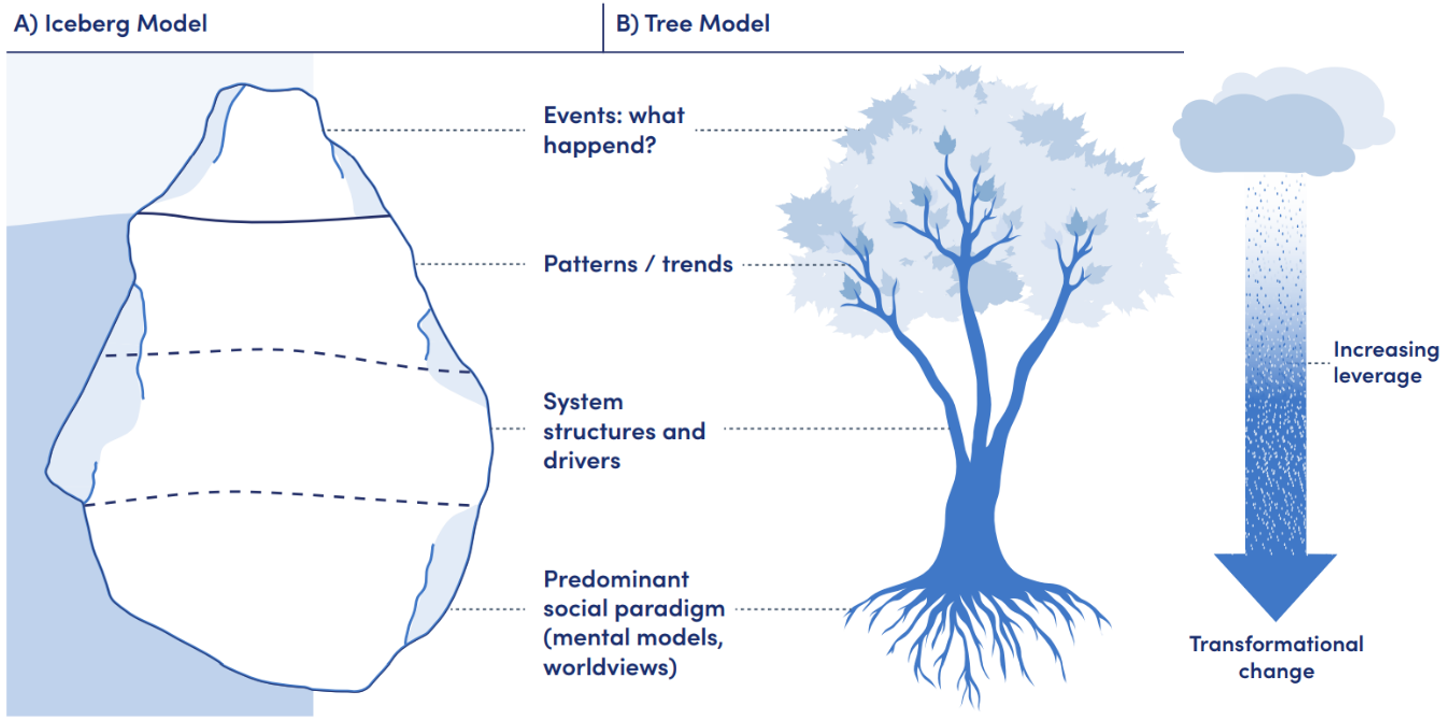
In sum, the value of systems thinking is that it can help us to understand the world around us. Many of the complex adaptive systems in our world are unpredictable, uncontrollable, and understandable only in general ways—but systems thinkers can see that there is a wide range of choices before us. They can study and understand the intricacies of systems, with their embedded subsystems and dynamic interrelationships. Systems can then be nudged or even or redesigned, and assumptions and trade-offs that can be anticipated and learned from.
Attribution: Betley, E., Sterling, E. J., Akabas, S., Paxton, A., & Frost, L. (2021). Introduction to Systems and Systems Thinking. Lessons in Conservation, 11(1), 9–25. Modified from the original by A. Geddes. See paper for more on this topic, including extensive resources list, further reading, and systems thinking tools and frameworks. Copyright 2021, by the authors of the material and the Center for Biodiversity and Conservation of the American Museum of Natural History. All rights reserved, used with permission.