1.2 Science is a Process
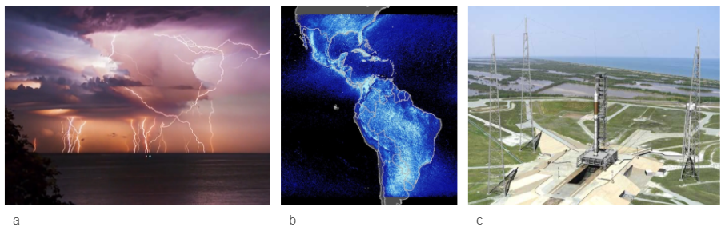
Ok, so Environmental Science is western science examining nature, the environment. But what is science exactly?
Science is fundamentally a process. It’s a way that humans have found works very well to gather knowledge about the natural world and figure things out. The methods of science include careful observation, record keeping, logical and mathematical reasoning, experimentation, and submitting conclusions to the scrutiny of others. Science also requires considerable imagination and creativity; a well-designed experiment is commonly described as elegant or beautiful.
And part of why we do science is because we like to figure things out, because there is a fascination and a satisfaction which comes from understanding how our world works and why things happen. Is lightning caused by imbalances in electric charge between two locations, or is it because Thor is angry? Well, if all you want is a story, then either one is just as good. But the main reason that science is so successful is because it works really well at giving us the knowledge and the paradigms needed to not only understand and appreciate our world, but also to make predictions about it. Understanding electrical potential and discharge allowed Benjamin Franklin to invent lightning rods, which in turn stopped houses from getting struck by lightning and burning down (figure 1).
Science has considerable practical implications and some science is dedicated to practical applications, such as the prevention of disease (figure 2). Other science proceeds largely motivated by curiosity. Whatever its goal, there is no doubt that science has transformed human existence and will continue to do so.
The Nature of Science
Science (from the Latin scientia, meaning “knowledge”) can be defined as a process of gaining knowledge about the natural world.
Science is a very specific way of learning about the world. The history of the past 500 years demonstrates that science is a very powerful way of gaining knowledge about the world; it is largely responsible for the technological revolutions that have taken place during this time. There are areas of knowledge, however, that the methods of science cannot be applied to. These include such things as morality, aesthetics, or spirituality. Science cannot investigate these areas because they are outside the realm of material phenomena, the phenomena of matter and energy, and cannot be observed and measured.
The scientific method is a method of research with defined steps that include experiments and careful observation. The steps of the scientific method will be examined in detail later, but one of the most important aspects of this method is the testing of hypotheses. A hypothesis is a proposed explanatory statement, for a given natural phenomenon, that can be tested. Hypotheses, or tentative explanations, are different from a scientific theory. A scientific theory is a widely-accepted, thoroughly tested and confirmed explanation for a set of observations or phenomena. Scientific theory is the foundation of scientific knowledge. In addition, in many scientific disciplines (less so in biology) there are scientific laws, often expressed in mathematical formulas, which describe how elements of nature will behave under certain specific conditions, but they do not offer explanations for why they occur.
Natural Sciences
What would you expect to see in a museum of natural sciences? Frogs? Plants? Dinosaur skeletons? Exhibits about how the brain functions? A planetarium? Gems and minerals? Or maybe all of the above? Science includes such diverse fields as astronomy, computer sciences, psychology, biology, and mathematics. However, those fields of science related to the physical world and its phenomena and processes are considered natural sciences and include the disciplines of physics, geology, biology, and chemistry. Environmental science is a cross-disciplinary natural science because it relies of the disciplines of chemistry, biology, and geology.
Scientific Inquiry
One thing is common to all forms of science: an ultimate goal to know. Curiosity and inquiry are the driving forces for the development of science. Scientists seek to understand the world and the way it operates. Two methods of logical thinking are used: inductive reasoning and deductive reasoning. We sometimes rely on only one, but often we use both of these in concert.
Inductive reasoning is a form of logical thinking that uses related observations to arrive at a general conclusion. This type of reasoning is common in descriptive science. A life scientist such as a biologist makes observations and records them. These data can be qualitative (descriptive) or quantitative (consisting of numbers), and the raw data can be supplemented with drawings, pictures, photos, or videos. From many observations, the scientist can infer conclusions (inductions) based on evidence. Inductive reasoning involves formulating generalizations inferred from careful observation and the analysis of a large amount of data. Brain studies often work this way. Many brains are observed while people are doing a task. The part of the brain that lights up, indicating activity, is then demonstrated to be the part controlling the response to that task.
Deductive reasoning or deduction is the type of logic used in hypothesis-based science. In deductive reasoning, the pattern of thinking moves in the opposite direction as compared to inductive reasoning. Deductive reasoning is a form of logical thinking that uses a general principle or law to forecast specific results. From those general principles, a scientist can extrapolate and predict the specific results that would be valid as long as the general principles are valid. For example, a prediction would be that if the climate is becoming warmer in a region, the distribution of plants and animals should change. Comparisons have been made between distributions in the past and the present, and the many changes that have been found are consistent with a warming climate. Finding the change in distribution is evidence that the climate change conclusion is a valid one.
Both types of logical thinking are related to the two main pathways of scientific study: descriptive science and hypothesis-based science. Descriptive (or discovery) science aims to observe, explore, and discover, while hypothesis-based science begins with a specific question or problem and a potential answer or solution that can be tested. The boundary between these two forms of study is often blurred, because most scientific endeavors combine both approaches. Observations lead to questions, questions lead to forming a hypothesis as a possible answer to those questions, and then the hypothesis is tested. Thus, descriptive science and hypothesis-based science are in continuous dialogue.
Hypothesis Testing
Environmental scientists study the living world by posing questions about it and seeking science-based responses. This approach is common to other sciences as well and is often referred to as the scientific method. The scientific method was used even in ancient times, but it was first documented by England’s Sir Francis Bacon (1561–1626) who set up inductive methods for scientific inquiry. The scientific method is not exclusively used by biologists but can be applied to almost anything as a logical problem-solving method.
The scientific process typically starts with an observation (often a problem to be solved) that leads to a question. Let’s think about a simple problem that starts with an observation and apply the scientific method to solve the problem. One Monday morning, a student arrives at class and quickly discovers that the classroom is too warm. That is an observation that also describes a problem: the classroom is too warm. The student then asks a question: “Why is the classroom so warm?”
Recall that a hypothesis is a suggested explanation that can be tested. To solve a problem, several hypotheses may be proposed. For example, one hypothesis might be, “The classroom is warm because no one turned on the air conditioning.” But there could be other responses to the question, and therefore other hypotheses may be proposed. A second hypothesis might be, “The classroom is warm because there is a power failure, and so the air conditioning doesn’t work.”
Once a hypothesis has been selected, a prediction may be made. A prediction is similar to a hypothesis but it typically has the format “If . . . then . . . .” For example, the prediction for the first hypothesis might be, “If the student turns on the air conditioning, then the classroom will no longer be too warm.”
A hypothesis must be testable to ensure that it is valid. For example, a hypothesis that depends on what a bear thinks is not testable, because it can never be known what a bear thinks. It should also be falsifiable, meaning that it can be disproven by experimental results. An example of an unfalsifiable hypothesis is “Botticelli’s Birth of Venus is beautiful.” There is no experiment that might show this statement to be false. To test a hypothesis, a researcher will conduct one or more experiments designed to eliminate one or more of the hypotheses. This is important. A hypothesis can be disproven, or eliminated, but it can never be proven. Science does not deal in proofs like mathematics. If an experiment fails to disprove a hypothesis, then we find support for that explanation, but this is not to say that down the road a better explanation will not be found, or a more carefully designed experiment will be found to falsify the hypothesis.
Each experiment will have one or more variables and one or more controls. Experimental variables are any part of the experiment that can vary or change during the experiment. Controlled variables are parts of the experiment that do not change. Lastly, experiments might have a control group: a group of test subjects that are as similar as possible to all other test subjects, with the exception that they don’t receive the experimental treatment (those that do receive it are known as the experimental group). For example, in a study testing a weight-loss drug, the control group would be test subjects that don’t receive the drug (but they might receive a placebo, such as sugar pill, instead). Look for these various things in the example that follows:
An experiment might be conducted to test the hypothesis that phosphate (a nutrient) promotes the growth of algae in freshwater ponds. A series of artificial ponds are filled with water and half of them are treated by adding phosphate each week, while the other half are treated by adding a non-nutritional mineral that is not used by algae. The experimental variable here is presence/absence of a nutrient (phosphate). One potential controlled variable would be the volume of water in each tank. The amount of water that algae have access to may influence the results, thus researchers want to control its influence on the results by making sure all test subjects get the same amount. The control group consists of the tanks that received a placebo (non-nutritional mineral) instead of the phosphate. If the ponds with phosphate show more algal growth, then we have found support for the hypothesis. If they do not, then we reject our hypothesis. Be aware that rejecting one hypothesis does not determine whether or not the other hypotheses can be accepted; it simply eliminates one hypothesis that is not valid (Figure 3). Using the scientific method, the hypotheses that are inconsistent with experimental data are rejected.
In the example below, the scientific method is used to solve an everyday problem. Which part in the example below is the hypothesis? Which is the prediction? Based on the results of the experiment, is the hypothesis supported? If it is not supported, propose some alternative hypotheses.
- My toaster doesn’t toast my bread.
- Why doesn’t my toaster work?
- There is something wrong with the electrical outlet.
- If something is wrong with the outlet, my coffeemaker also won’t work when plugged into it.
- I plug my coffeemaker into the outlet.
- My coffeemaker works.
In practice, the scientific method is not as rigid and structured as it might at first appear. Sometimes an experiment leads to conclusions that favor a change in approach; often, an experiment brings entirely new scientific questions to the puzzle. Many times, science does not operate in a linear fashion; instead, scientists continually draw inferences and make generalizations, finding patterns as their research proceeds. Scientific reasoning is more complex than the scientific method alone suggests.
Real-world Science
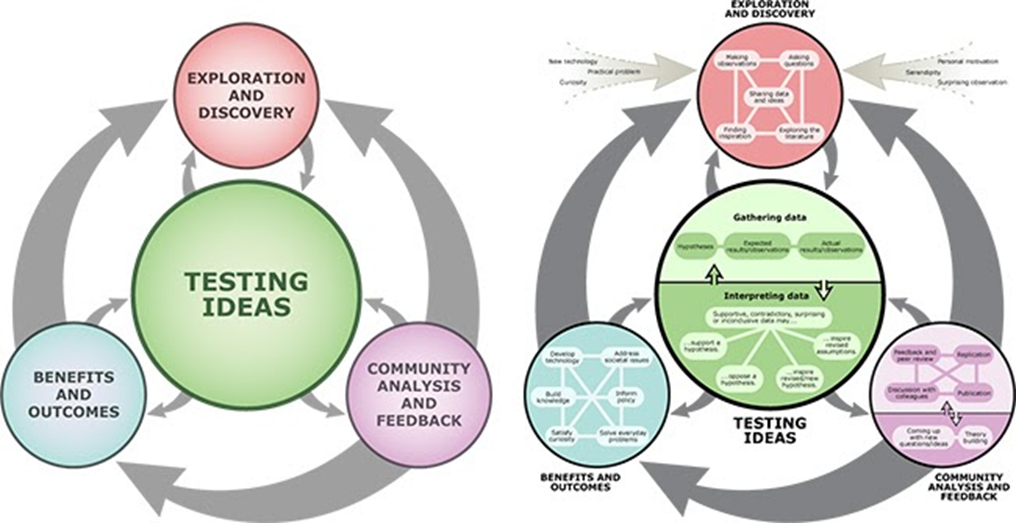
In practice, the scientific method is not as rigid and structured as it might at first appear. In fact, the process is always iterative, and often messy, and is influenced by the many interactions and influences that a person experiences, personally and professionally (Figure 5). Scientists continually draw inferences and make generalizations, finding patterns as their research proceeds. Sometimes an experiment leads to conclusions that favor a change in approach. Often, an experiment brings entirely new scientific questions to the puzzle. The benefits to your society, or the funding that someone is willing to give you, might push you to explore one set of questions over another. And most science involves a lot of missteps.
And of course scientists work within a community, we are always adding to and drawing from the knowledge of everyone else who has studied the problem before. This might be published papers in scientific journals, or just chatting with colleagues. Sir Isaac Newton (calculus, the three basic laws of motion) famously wrote “if I have seen further, it is by standing on the shoulders of giants,” which is to say that by using the understanding gained by those who have gone before us, we can make greater intellectual progress.
Curious to read more?
This article about the origins of the mRNA vaccines is an excellent account of how real world science works. Curiosity, greed, funding, politics, bias, collaboration, immigrants, and a chance meeting at a copy machine.
The excellent website Understanding Science, where figure 5 comes from.
Experimental Methods
Not only is scientific reasoning more complex than the scientific method alone suggests, but also how we test ideas, what we mean by an “experiment”, can look very different depending on what question you are asking. This can be particularly true in environmental science, because so many of the processes and systems that we’re studying are too big, too complex, or take place over too long of a time period to be reproduced in a lab. How do volcanoes impact climate? What conditions limit or encourage regrowth of forests after fires? Are populations of jaguars in different parts of Venezuela interbreeding? These are questions which you can’t answer with a controlled experiment in a lab, because you can’t recreate the conditions you are exploring.
A controlled experiment will set up multiple sets of samples or subjects, and then compare the results. They will have a control group or control sample, as described above. The experimenter will do everything they can to make all the conditions for all the groups or samples exactly the same (controlled), except for the one variable which they are testing. So for example, maybe you want to know if fluoride in water helps seeds germinate. Well, you could set up a controlled experiment to test this, with several groups of seeds that are treated exactly the same, are sprouted under the same conditions except that some are given water with various concentrations of fluoride (the treatment) while one group is given none (the control).
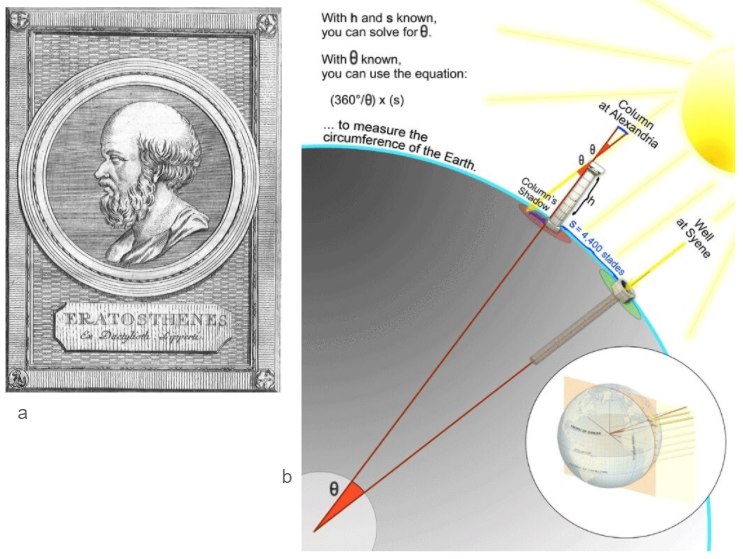
Erastothenes measured the angle 𝜃 to be 1/50 of a circle and his access to knowledge of the size of Egypt gave a north-south distance s between Alexandria and Syene. His calculated circumference of the Earth had an error of less than 6% from the true value for the polar circumference! Credit: NOAA Ocean Service Education
The term “experiment” usually implies a controlled experiment to most people. But in the natural science, and in environmental science in particular, we are often studying complex systems, with infinitely intricate webs of interactions and interrelationships. So we often use natural experiments. In these studies we rely on observations of the variables we are interested in within the systems themselves, or out in nature. Instead of manipulating one variable ourselves, we would look for existing locations where that particular condition varies. The problem is that in different places there might be more differences than just the one variable we’d like to test. Water fluoridation levels differ widely in nature, so we could easily find places where the plants are exposed to none, or to lots. But the difficulty is that those places also might have more or less sunlight, they might have very different soil chemistry, or there might be birds or mice eating the seeds before they are able to germinate.
So why do we use natural experiments? Because there are some experiments we can’t do in a lab. The North African scientist and mathematician Eratosthenes calculated the size of the Earth in 240 BCE (people there knew it was round at that time) by comparing the angle of sunlight at different places on the planet. These measurements needed to be done in the real world environment (figure 6).
Observational studies are also sometimes done in cases where it would be unethical or impractical to artificially produce the treatments you want to study. This is often the case in public health, for example. If we are concerned that high voltage power lines might be dangerous to people, then we wouldn’t ask them to expose themselves just for our experiment. Also, we are interested in long term exposures. So instead we might simply map the distances from various houses to the nearest high voltage lines, and compare those values to health indicators in the people who live there.
![[Person is pointing at a line graph at a specific point where it slopes down. The y-axis shows that as y increases, love increases.] Person: Our relationship entered its decline at this point. [Outside of panel]: That's when you started graphing everything. Person: Coincidence!](https://openoregon.pressbooks.pub/app/uploads/sites/262/2017/03/image_2022-01-03_021449.png)
In general, natural or observational experiments only tell us that there is a correlation between variables, they don’t prove causation. If two things are correlated, then they move in coordination with each other. But that doesn’t prove that one causes the other. For example, let’s say you compared the number of bars in various cities, with the number of art galleries. While there will be variation, you would find in general that cities with lots of bars also have lots of art galleries, while cities with only one or two bars probably only have at most a few art galleries. Does this mean that bars are necessary for the creation of art galleries? Or that only drunk people buy art? No, there is a confounding factor in this data, something which you haven’t measured but which is driving both of your variables: the total population! In a small town, you won’t have very many bars OR art galleries, but in bigger cities you’ll have more of both. Correlation does not imply causation.
In addition to these types of field or lab work, a lot of cutting edge science also used models to test hypotheses. These might be physical scale models, of processes or objects which are very large or very small. But more often today we would use digital models. Scientists take all of the information that we already know about the interrelationships among the various elements in a system, and program them into a model of the system as a whole. They can test the model by running it with real world conditions, and see if it produces the same results as what were seen in the real world system. For example, we could model how groundwater moves through a hillside, based on what we think we know about the soil, topography, and vegetation there. Then we could test our model by giving it the amount of rainfall that actually fell, and measuring the streamflow it predicts should have come out at the bottom of the hill. If we create a model which accurately models real world processes, then we can change the inputs and parameters of our model to test how those changes might affect things. We could change the steepness of the hill in our model, which would be impossible to do in the real world.
Basic and Applied Science
Is it valuable to pursue science for the sake of simply gaining knowledge, or does scientific knowledge only have worth if we can apply it to solving a specific problem or bettering our lives? This question focuses on the differences between two types of science: basic science and applied science.
Basic science or “pure” science seeks to expand knowledge regardless of the short-term application of that knowledge. It is not focused on developing a product or a service of immediate public or commercial value. The immediate goal of basic science is knowledge for knowledge’s sake, though this does not mean that in the end it may not result in an application.
In contrast, applied science aims to use science to solve real-world problems, such as improving crop yield, finding a cure for a particular disease, or saving animals threatened by a natural disaster. In applied science, the problem is usually defined for the researcher.
Some individuals may perceive applied science as “useful” and basic science as “useless.” It is true that there are problems that demand immediate attention; however applied science often relies on the results generated through basic science. One example of how basic and applied science can work together to solve practical problems occurred after the discovery of DNA structure led to an understanding of the molecular mechanisms governing DNA replication. This basic science enabled scientists to develop laboratory techniques that are now used to identify genetic diseases, pinpoint individuals who were at a crime scene, and determine paternity (all examples of applied science) (Figure 7).
Scientific Work is Transparent & Open to Critique
Whether scientific research is basic science or applied science, scientists must share their findings for other researchers to expand and build upon their discoveries. For this reason, an important aspect of a scientist’s work is disseminating results and communicating with peers. Scientists can share results by presenting them at a scientific meeting or conference, but this approach can reach only the limited few who are present. Instead, most scientists present their results in peer-reviewed articles that are published in scientific journals. Peer-reviewed articles are scientific papers that are reviewed, usually anonymously by a scientist’s colleagues, or peers. These colleagues are qualified individuals, often experts in the same research area, who judge whether or not the scientist’s work is suitable for publication. The process of peer review helps to ensure that the research described in a scientific paper or grant proposal is original, significant, logical, ethical, and thorough. Scientists publish their work so other scientists can reproduce their experiments under similar or different conditions to expand on the findings. The experimental results must be consistent with the findings of other scientists.
As you review scientific information, whether in an academic setting or as part of your day-to-day life, it is important to think about the credibility of that information. You might ask yourself: has this scientific information been through the rigorous process of peer review? Are the conclusions based on available data and accepted by the larger scientific community? Scientists are inherently skeptical, especially if conclusions are not supported by evidence (and you should be too).
Suggested Supplementary Reading:
Sundermier, A. 2016. “These 5 mind-melting thought experiments helped Albert Einstein come up with his most revolutionary scientific ideas.” Business Insider. <https://www.businessinsider.com/5-of-albert-einsteins-thought-experiments-that-revolutionized-science-2016-7>
Attribution
Concepts of Biology by OpenStax is licensed under CC BY 3.0. Modified from the original by A. Geddes and Matthew R. Fisher.