8 Tooth development
- Overview
- Crown development
- Initiation stage
- Bud stage
- Cap stage
- Bell stage
- Crown stage
- Root development
- Root dentin formation
- Cementum & pulp formation
- Periodontal ligament
- Alveolar bone
- Tooth eruption
- Clinical applications
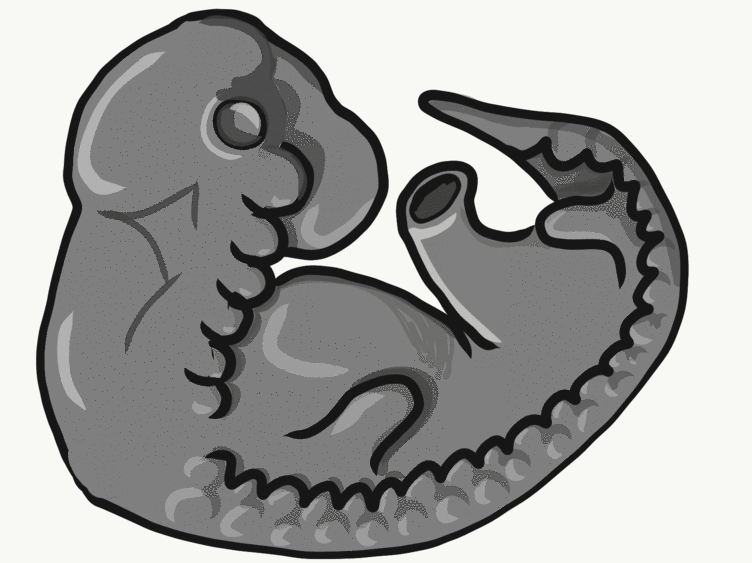
Overview of tooth formation
In this chapter we cover major concepts in tooth development, saving many details for later chapters. The first big concept is the timing: so far everything has been measured in weeks, but in this chapter we measure time in weeks, months and years. Both the primary and succedaneous teeth begin developing early in embryonic development—around week 5. To put this in perspective, that is only shortly after the mouth forms– the oro-pharyngeal membrane ruptures in week 4. The process of tooth development begins around week 5. When tooth development ends is more complicated, as listed in Table 8.1 (note: any timeline you see most likely reports an average or most common age, there is significant variation in the timeline between individuals).
Tissue: | Develops during: |
---|---|
crown enamel and dentin – primary | embryonic week 5 to 8 |
crown enamel and dentin – succedaneous | embryonic week 8 to after birth (5 years) |
root dentin and cementum – primary | embryonic to after birth (1 – 3 years) |
root dentin and cementum- succedaneous | after birth (6 – 25 years) |
PDL, alveolar bone, junctional epithelium← | tooth eruption |
The next big concept is the importance of the very first stage (placode, or induction). Small regions of epithelial cells in the oral mucosa are induced to speed up mitosis←. This happens because of secretion of morphogens← from neural crest cells← below. You can’t see this under the microscope (not without more specialized tools than the H&E stain). As a result, this early phase is often skipped in histology books. That is a shame, because getting the correct number of teeth to form requires complex communication. Not only do the correct number need to form, the spacing between succedaneous teeth depends on where primary teeth develop. To put that another way, the embryo is making plans 5 weeks after fertilization for events that happen 5 to 10 years later. To put it yet another way, when the embryo is about 1.5 mm in length (about 1/16th of an inch), it is making plans for the spacing of teeth in an adult skull that will be 150 mm across (about 6 inches). We think this early communication is really cool. There are, however, more names associated with later stages (usually named after long-dead European men), and those names appear on licensing exams, so we cover those in detail as well. Lucky you.
The third big concept is the lineage of the different parts of a tooth and periodontium. Enamel is produced by cells derived from the ectoderm, whereas dentin, cementum, pulp, PDL and alveolar bone are derived from neuro-mesenchyme. While the hard tissues enamel, dentin and cementum share physical characteristics with bone tissue←, enamel has notable differences because of its different lineage. Perhaps the biggest difference is that people cannot grow new enamel after teeth erupt. Enamel can passively re-mineralize under the right conditions, but this does not involve the activity of human cells, scaffolds← or enzymes (which are more efficient). The enamel-producing cells, including the scaffolds and enzymes they secrete, are lost during tooth eruption. In contrast, dentin, pulp, cementum and the PDL contain cells throughout the life of a tooth, and have a much higher capacity to undergo remodeling and regenerate following trauma.
The fourth big concept is that the mesenchyme← is worthy of attention. We did not say mesoderm (one of the three embryonic germ layers), but mesenchyme (the tissue type)—the difference is important. Most mesenchyme is derived from mesoderm, but tooth connective tissues are derived from neural crest cells← plus mesoderm. We give this mesenchyme a unique name: neuro-mesenchyme. This leads to similarities in the behavior of tooth and brain cells, which makes less sense to people who haven’t learned embryology.
The fifth big concept is that enamel and dentin-producing cells do not form separately. They induce one another in a reciprocal fashion. After their team-effort, the cells move apart from one another and do their own thing. This reduces the chances that enamel or dentin will be produced in the wrong place or wrong time. Production of these tissues requires a special set of conditions, one that occurs only briefly during early embryonic development. Unfortunately, this makes it harder for medical professionals to re-create these conditions later in life should enamel or dentin production be beneficial.
The sixth and final big concept is that the process of tooth eruption is complicated. There are multiple steps which are poorly understood, and scientists disagree on which steps are important. We cover what is known, but we can let the experts keep arguing. What is important to us is that to learn about the development of the PDL, alveolar bone and junctional epithelium←, we must cover tooth eruption. Tooth eruption should be considered a developmental process even though it happens long after the embryonic and fetal stages.
Crown development

"Cameriere's method of measurement for the teeth with open apices"
by Navaneetha Cugati, Ramesh Kumaresan, Balamanikanda Srinivasan, Priyadarshini Karthikeyan is licensed under CC BY-NC 3.0Tooth formation, or odontogenesis, starts with primary teeth around weeks 5 to 6, and succedaneous teeth around weeks 9 to 10. Odontogenesis is similar to neurulation←, teeth arise from invaginations of epithelium, derived from ectoderm. Hair follicles share this pattern as well, but instead of being called a tooth follicle, teeth grow from tooth buds. At week 5, the oral cavity is lined by ectoderm-derived epithelium, and deep to that is mesenchyme←. For the skin and most of the oral cavity, the layer of mesenchyme is derived from mesoderm. Teeth, however, grow from ridges called the dental lamina, found on the mandibular arch and the maxillary processes, along the occlusal border. Here, mesenchyme contains clusters of neural crest cells← that migrated from the neural tube. Hence, we say the connective tissues of the teeth are derived from neuro-mesenchyme (or ecto-mesenchyme). Remember, because neural crest cells come from the neural tube, and the neural tube invaginates from the ectoderm, these cells are ectodermal by lineage. They go through an epithelial-to-mesenchymal transition← and resemble mesenchyme derived from mesoderm under the microscope. If you remember how cells express matrix metalloproteinase enzymes during this transition, you shouldn’t be surprised to see these enzymes re-used during odontogenesis, and re-used yet again during tooth eruption.
By 8 weeks (before the palate fuses), there are 10 tooth buds in each dental lamina (the regions fated to become the maxillary and mandibular alveolar ridges). The tooth buds of succedaneous teeth begin forming around this time, before the primary buds have made any enamel or dentin. Both primary and permanent buds develop into crowns and become surrounded by the maxilla and mandible (Fig. 8.2).

“Signaling pathways in placode formation"
by Andrea Streit, Stembook.org
is licensed CC BY 3.0Induction (or initiation)
The first step is poorly understood. Neuro-mesenchymal stem cells coordinate with one another through planar cell polarity← signals to induce the correct number of tooth buds, at the correct distance apart from one another. Neuro-mesenchymal stem cells secrete a number of morphogens← forming complex gradients. Overlying ectoderm responds to the morphogen gradients.

Two important morphogens← in tooth development are members of the FGF and the BMP families of signaling molecules. There are more signals involved, which is illustrated in Fig. 8.3 (further reading
). As you might guess from their full names, BMP and FGF aren’t unique to tooth formation. These two morphogens induce different cells to do different things at different times and locations. At this time and location, they induce ectoderm to differentiate← into tooth buds instead of oral mucosa. Other morphogens inhibit tooth bud induction, ensuring proper spacing is established. One of the first visible responses in the ectoderm is proliferation— certain regions begin to grow thicker, called tooth placodes. Not every book (or exam) includes placode as its own stage, be aware. Furthermore, placodes may be called the dental lamina or may be listed as a part of the dental lamina—fate mapping experiments
can’t easily be done on humans to determine which is more correct. The important concept is most of the oral mucosa described in chapter 3 is derived from ectoderm and mesoderm, while the teeth and periodontium are derived from ectoderm and the neuro-mesenchyme of a tooth bud.
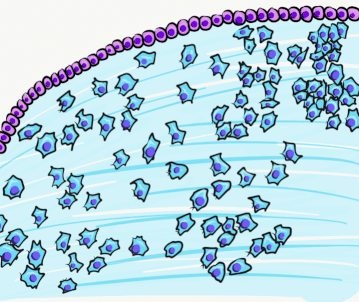
Bud stage
Continued proliferation of the ectoderm allows us to see the next stage of tooth development under the microscope more easily, the bud stage. The name bud comes from the fact that tooth buds look like leaf buds on a plant. In the spring, you can see where leaves are fated to grow on plants by the location of leaf buds. Leaf buds are not leaves yet, just bumps. Tooth buds begin to appear around week 6, and ultimately 10 tooth buds form on the maxillary processes and mandibular arch. In addition to proliferation of ectodermal cells, neuro-mesenchymal stem cells also proliferate. This makes the mesenchyme← in a tooth bud appear denser than the mesenchyme in nearby regions that form the lamina propria of the oral mucosa. Those regions have more of the mucous ground substance← and fewer cells.
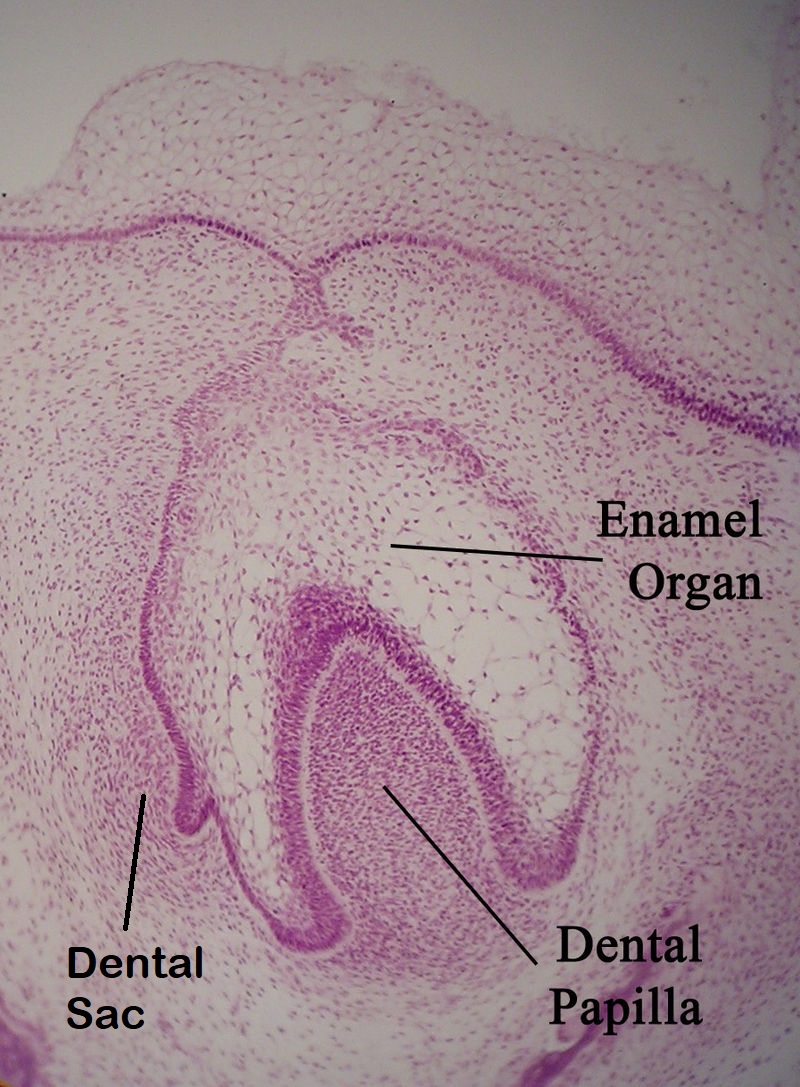
Enamel organ
“ by Dozenist is licensed under CC BY-SA 3.0Cap stage
Around week 10, the ectoderm continues to proliferate and it bumps into the cluster of neuro-mesenchymal stem cells below. This cluster of neuro-mesenchymal stem cells is called the dental papilla. The dental papilla forces the ectoderm of the tooth bud to grow around it. As this happens, the ectoderm resembles a hat, hence the name cap stage. At this time, the dental lamina has two prominent parts—an inner and an outer layer. The epithelial cells near the dental papilla are referred to as the enamel organ, because some of them differentiate← into cells that produce enamel. Below the dental lamina, the neuro-mesenchyme of the dental papilla forms dentin and pulp. The neuro-mesenchyme on the outside of the dental lamina is called the dental sac (or dental follicle), which forms cementum, PDL and alveolar bone. All three together—the enamel organ, dental papilla and dental sac—are collectively called tooth germ.
At this time, the process repeats (or recapitulates). Cells of the dental lamina on the lingual side of the cap are induced to form another placode, and the succedaneous teeth bud off the developing primary tooth germ. Imagine taking Fig 8.5, rotate it 90o, superimpose that onto the cap stage, and you have succedaneous tooth bud formation. Exceptions to this include the 2nd and 3rd molars, which do not succeed primary teeth. Their tooth buds develop from ectoderm similar to the primary dentition. Furthermore, the tooth germ of succedaneous teeth can move as they develop—tooth eruption does not always occur from the lingual side. For instance, the maxillary incisors generally erupt from the facial direction.
Bell stage

By the 11th or 12th week, ectoderm and neuro-mesenchyme continue to proliferate. As the epithelial cap becomes larger, it resembles more of a bell shape, hence the name bell stage. Hats worn by people are usually smaller than bells on clock towers, which may help you remember which stage comes before the other. At this time, the enamel organ contains two layers of cuboidal cells named the inner enamel epithelium (IEE) and the outer enamel epithelium (OEE). The IEE is next to the dental papilla, with a basement membrane physically separating the two. The IEE differentiates← into ameloblasts and produce enamel, while the OEE is involved in tooth eruption and forms the junctional epithelium← that bridges the tooth surface and oral mucosa.

Two additional regions of the enamel organ develop at this time. The stratum intermedium is on the opposite side of the IEE from the dental papilla. These cells work with the IEE to form enamel. Past the stratum intermedium are ectodermal cells called stellate reticulum, named for their star-shaped rather than cuboidal appearance. These cells help induce the IEE to differentiate← and begin enamel production. These cells are found in the superficial but not the deeper regions of the growing tooth germ, which is why enamel is only produced in crowns and not in roots of teeth.
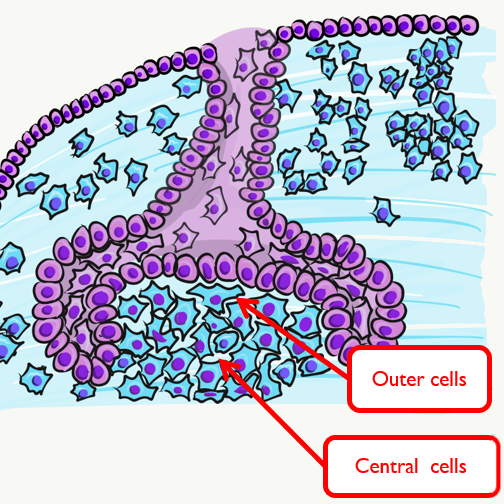
The dental papilla may be subdivided at this time into an outer region and a central region. The outer cells differentiate← into odontoblasts, while the central cells form many cell types found in pulp. It is worth noting that cells are not ameloblasts or odontoblasts at this time. These cells are epithelial and neuro-mesenchymal stem cells. Based on their relative location, we know what they are fated to become in the near future, assuming they receive the correct morphogens←.
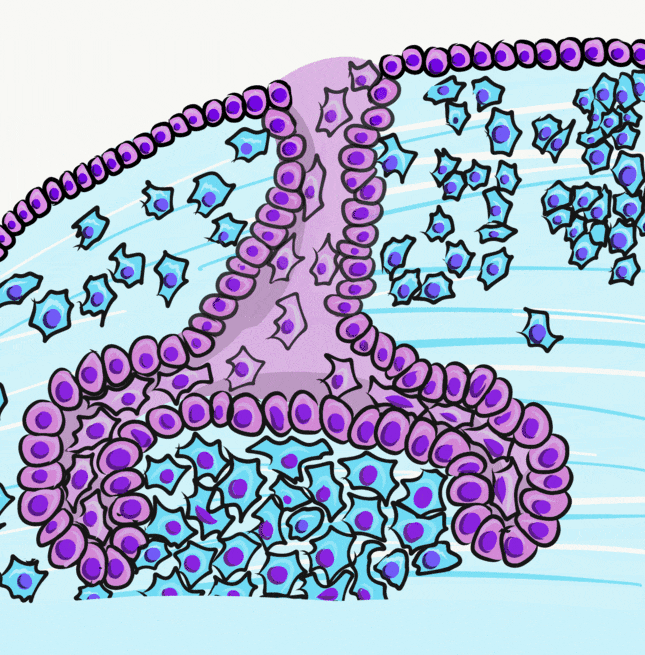
A brief overview of the formation of ameloblasts and odontoblasts
During the bell stage, interactions between the IEE, stellate reticulum and outer cells of the dental papilla induce the differentiation← of ameloblasts and odontoblasts. First, the basement membrane that physically separated the IEE from the neuro-mesenchymal stem cells of the dental papillae disintegrates. The IEE contacts ECM made by neuro-mesenchymal stems cells, am=nd they differentiate into pre-ameloblasts. The prefix pre- indicates that differentiation is not considered complete at this time. The IEE looks different: cells elongate, changing from a simple cuboidal epithelium← to a simple columnar epithelium←. Pre-ameloblasts then produce morphogens← that induce neighboring neuro-mesenchymal cells to differentiate into odontoblasts (the cells that make dentin). Newly formed odontoblasts begin producing a squishy immature form of dentin (pre-dentin). Molecules in pre-dentin signal back to the pre-ameloblasts. Morphogens from the stellate reticulum also signal to pre-ameloblasts. The combination of these 2 signals induces the differentiation of pre-ameloblasts into ameloblasts (the cells that make enamel).

This is a common pattern in developmental biology. A dual effort is known as reciprocal induction
. This is significant because it makes it hard to re-create one type of cell later in life (such as an ameloblast) without also re-creating its partner (in this case, an odontoblast). Furthermore, one must also re-create the conditions these cells were in during reciprocal induction (in this case, being close to stellate reticulum).
Dentinogenesis, the formation of dentin, therefore begins before amelogenesis, the formation of enamel. This results in dentin being thicker than enamel. As odontoblasts secrete pre-dentin, their cell bodies are pushed deeper, but they leave a log tube-like extension called the odontoblastic process within the pre-dentin. By the time a tooth is done growing, the odontoblastic processes extend through nearly the entire layer of mature dentin. Ameloblasts, on the other hand, do not grow an extension. There is a little bump on each ameloblast facing the enamel, and this bump is called Tomes' process. There is important clinical significance related to odontoblastic processes coming up in subsequent chapters. Tomes’ process, on the other hand, is a name of a bump histologists use to identify ameloblasts during embryonic development (and a name prospective dental hygienists get asked about on license exams).
Crown stage
The second half of the bell stage is the late bell stage, or the crown stage. During this time the crowns of teeth form by the secretion of molecules by ameloblasts and odontoblasts. No new cells of these types form, teeth continue to grow by the addition of ECM. Because the ECM mineralizes and hardens, these tissues must be added appositionally. This is somewhat similar to the growth of bone tissue←, only the enamel and dentin-producing cells do not get trapped between layers of hard tissue. Instead, these cells migrate in a basal direction (relative to the cell) as they secrete matrix. Its important to note the process of crown formation finishes before mineralization of the mandible and maxilla, but mineralization of the crowns takes more time, finishing within the first year or two of life. The timeline for succedaneous teeth is more variable (a timetable may be found on wikipedia
).
Root Development

Latebellstage11-18-05
"
by Dozenist
is licensed under CC BY 3.0 / Iabels addedThe cervical loop and HERS
We must jump ahead in time to cover root development: months for primary teeth, and years for succedaneous teeth. After the crown stage, the leading edge of the IEE and OEE continue to grow around the dental papilla. However, they are not separated by stellate reticulum. Together they are called the cervical loop. As the cervical loop continues to grow deeper, it is called Hertwig’s Epithelial Root Sheath (HERS). The pattern of HERS growth determines the shape of the root(s). This is similar to a cake mold: HERS does not become the roots, it guide their shape. HERS does not grow evenly around the dental papillae of larger teeth. Instead, HERS grows faster in some regions as it extends over the dental papilla, perhaps the way chocolate fudge drips over a ball of ice-cream unevenly. On larger teeth, this produces multiple roots. The cervical loop forms early in embryonic development, but HERS continues to grow after birth. The roots wont completely mineralize until years after tooth eruption.
Cementum formation
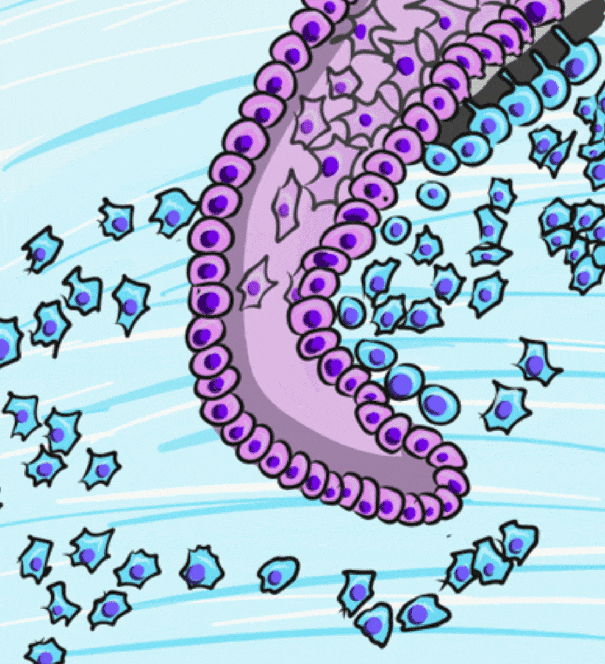
HERS contains IEE and OEE, but no stellate reticulum. This leads to key differences between the crown and roots of teeth. The IEE induce the formation of odontoblasts in the roots just as it did in the crown, but when odontoblasts form pre-dentin, the lack of a signal from stellate reticulum means the differentiation← of ameloblasts does not occur. Hence, roots do not contain enamel. Instead, most of the IEE and OEE cells undergo apoptosis←. A few are left behind, which get the name the Epithelial Rests of Malassez (ERM). These leftover cells may have functions in the regeneration of damaged root tissues, although this is not currently well understood.
After the majority of the epithelial cells are removed, neuro-mesenchymal stem cells of the dental sac contact pre-dentin. Getting closer to BMP morphogens secreted by odontoblasts induces them to differentiate← into cementoblasts. Cementoblasts then cover the root dentin in a relatively thin layer of cementum. At first, the cementum produced is pure ECM. Because it lacks cells it is called acellular cementum. Later, in the apical root regions, cementoblasts become trapped within the ECM they secrete. This is cellular cementum. The cells are called cementocytes once trapped within lacunae. The presence or absence of cells is easy to identify under the microscope. However, it is the polarity of collagen← fibers in the ECM that is more important clinically. This will be covered in Chapter 11.
Tooth eruption
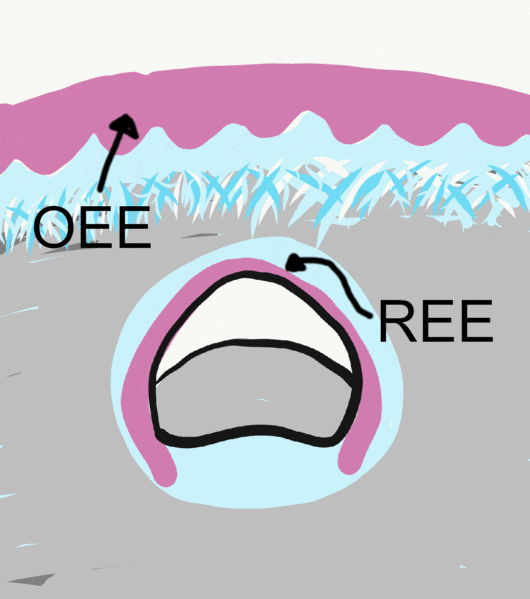
We now jump forward in time yet again. Even though process of tooth eruption happens long after embryological development, it should be considered a developmental process. Another way to say that is the teeth continue to undergo morphogenesis after birth. Because this process involves cells of the IEE and OEE, we discuss it here.
After the roots develop, neuro-mesenchymal stem cells of the dental sac that did not come into contact with pre-dentin differentiate← into fibroblasts and osteoblasts. These form the PDL and alveolar bone. The PDL anchors to cementum first, then to alveolar bone after eruption. Therefore, development of the PDL occurs during tooth eruption, much later than the formation of dentin and enamel.
First, the force or forces that cause teeth to erupt are not agreed upon
. It is not even clear if it is a pushing or a pulling force, or a combination of the two. Whatever the cause or causes of tooth eruption are, movement of the crowns into the oral cavity is known as active eruption. One might imagine that as a root grows deeper and bumps into the edge of a bony socket, further lengthening of the root would force the crown upwards. This would be similar to jumping: with your legs bent and feet on a hard floor, rapid extension of your legs pushes you upwards. This is known as the root formation theory
. To say this causes tooth eruption, it has to be both necessary and sufficient (a phrase commonly used in biological research). Because root-less teeth can erupt
suggests root growth is not necessary for eruption.
We are going to use the terms necessary and sufficient a few more times. Before we do, here is a simpler scenario: after you stop your car at a red light, to get the car moving again requires taking a foot off the brake, applying a foot to the gas pedal and having gas in the gas tank. All three are necessary, but none of them is sufficient to move your car through the intersection.
We know bone remodeling is necessary. Bone remodeling involves coordinated activity between osteoblasts and osteoclasts (the remodeling unit). However, tooth eruption is a different than the dynamic equilibrium of bone tissue. During tooth eruption, both osteoclasts and osteoblasts are active. However, they do not work togther as part of the remodeling unit, instead they work at different locations. As a result, equilibrium is not maintained. Bone tissue is removed above the tooth, but added below the tooth. We covered evidence for this when we discussed how permanent teeth fail to erupt in people with cleido-cranial dysostosis←. There, a faulty matrix metalloproteinase enzyme lead to the inability to degrade ECM proteins, leaving permanent teeth trapped within their bony crypt. This suggests bone remodeling is necessary, but not necessarily sufficient.
For the primary teeth, as growing roots encounter mineralized bones tissue below, hydrostatic pressure is put on the cells of the enamel organ. In response, the stellate reticulum secretes morphogens← that induce bone resorption. One morphogen is RANKL, a morphogen that induces osteoclast differentiation
. Another is Parathyroid Hormone
(PTH), which is called a hormone when it is secreted into the bloodstream. Here, it is secreted into the ECM, only stimulating osteoclast activity near the enamel organ. Because stellate reticulum is at the surface of enamel, these signals induce the formation of a pathway above the tooth, allowing it to erupt.
Clearing a path is necessary for tooth eruption, but it does not cause the tooth to move. As roots grow, they put pressure on bone tissue in the alveolar sockets below. Pressure on bone tissue triggers release of BMPs which leads to the deposition of bone tissue, pushing teeth outwards. Bite forces also contribute to BMP secretion. As we mentioned earlier, root growth is not necessary, but BMP secretion is necessary. That doesn’t really make sense, does it? We must be missing some critical information.
We know root growth is sufficient to induce BMP secretion, and BMP secretion is necessary for tooth eruption. However, there may be something else that can stimulate BMP secretion. That is our mising piece of critical information. Further evidence that pressure is necessary comes from observation that teeth fail to erupt in the absence of healthy PDL. In fact, pressure created by fibroblasts of the PDL may trigger the stellate reticulum to secrete morphogens← that trigger osteoclast differentiation← as well as bone tissue to release BMPs. Fibroblasts not only secrete collagen←, they remodel it as well. Shortening of collagen fibers anchored to cementum would pressure on both the tooth root and alveolar socket. So does this happen? Polarization of PDL fibroblasts is observed before tooth eruption, suggesting that remodeling of PDL collagen fibers inserted in cementum may actively pull the tooth outwards. It is currently unknown whether this involves planar cell polarity← morphogens, signals which have been well established in their role in polarizing epithelial cells. It seems possible, given the lineage of PDL fibroblasts: neuro-mesenchyme (which means ectoderm, the same lineage as highly polarized keratinocytes and ameloblasts).
But we are still left with a major question: how can the PDL be necessary for tooth eruption if a rootless tooth can erupt? Can a rootless tooth have PDL? Lets refresh what we know about the PDL. The PDL develops from the remaining neuro-mesenchymal stem cells after receiving a signal from the dental papilla. We know it is not the BMP signal that induces neuro-mesenchymal stem cells to develop into odontoblasts, cementoblasts or osteoblasts. We know the PDL first connects to cementum during eruption, and connects to alveolar bone after eruption. It seems unlikely that the PDL could develop on a rootless tooth, and even if the PDL did develop, it would have nothing to attach to. So next, let’s summarize what we know about tooth eruption:
Action | Reaction | Neccessary or sufficient for tooth eruption? |
root growth | pushes down (against alveolar socket) | not necessary* |
triggers BMP secretion in alveolar socket
triggers PTH and RANKL secretion from stellate reticulum |
necessary | |
unknown factor(s) | ||
PTH and RANKL secretion | clears a pathway above tooth | necessary |
BMP secretion | bone deposited in alveolar socket | necessary |
bone deposited in alveolar socket | pushes up (against tooth root) | necessary |
PDL attaches to cementum | pulls tooth from above | necessary |
Now that we have summarized the facts we have, it is a good time to re-visit our troublesome assumption: teeth can erupt without roots. When professionals report the eruption of rootless teeth, do they mean these teeth never formed roots, or did the roots undergo resorption during eruption? Sadly, waiting until we see the eruption of a rootless tooth is too late to answer this question. We need time-travel, psychic abilities, or lots of radiographs of unerupted teeth. Furthremore, it is difficult to make a strong conclusion that root growth is not necessary when the original report of rootless tooth eruption is missing
. No wonder the force of tooth eruption are not agreed upon. Luckily, we do not need to solve the controversy here. But it does bring up an important concept: when things don’t make sense, question your assumptions! Here is a good article on how and why:
Don’t be disappointed if science raises two questions for every question it answers. Let’s recapitulate our car metaphor as we summarize what we know about tooth eruption. The best evidence at this time is that a foot must be lifted off the brake pedeal (RANKL and PTH clear a pathway in bone tissue above), a foot must be placed on the gas pedal (BMP causes bone deposition in the alveolar sockets and pushes teeth from below) and there must be gasoline in the gas tank (the PDL pulls teeth outwards).

"The JE attached to the bioengineered tooth was derived from the odontogenic epithelium."
by Sara Yajima-Himuro, et al is licensed under CC BY 3.0But wait, there’s more. Changes in the gingiva must also occur for tooth eruption to proceed. Remodeling of oral mucosa after active eruption is known as passive eruption. Old tissue is removed to clear a path for the tooth to erupt. Teeth do not just tear their way through the gums. But do not think of this like digging a tunnel for cars to drive through. The first difference is oral mucosa is connected to the tooth near the CEJ as it erupts. Otherwise, oral bacteria could enter the sub-mucosa and cause gingivitis. The second difference is there is no tunnel. Bone tissue is added behind teeth as they erupt. So think of this like digging a lacuna for just one car, filling the lacuna behind the car as it travels, and trapping the car just as its front end clears the other side.
Before eruption begins, the crown of the tooth is topped with 2 layers of epithelial cells, ameloblasts and OEE. Collectively, these two layers of epithelial cells are called the Reduced Enamel Epithelium (REE). Like the invagination and separation of ectodermal cells during neurulation←, the REE becomes separated from the oral epithelium during dentinogenesis. During tooth eruption, the REE re-joins the oral epithelium. As the REE fuses it re-forms desmosomes← and pairs up CAMs← with oral epithelial cells, but maintains contact with enamel. Many REE cells undergo apoptosis←, but those that remain become the junctional epithelium←. This special epithelium maintains hemi-desmosome contacts on both sides of the tissue: apical (enamel) and basolateral (lamina propria).
Some of the REE may not fuse with the oral epithelium nor undergo apoptosis←, and remain partially stuck to the surface of a newly erupted tooth. This is known as the primary enamel cuticle (or the more old-fashioned name Nasmyth’s membrane). It can easily be removed by gentle brushing or mastication.
Because ameloblasts in the REE are lost with tooth eruption, no new enamel is produced by human cells afterwards. Amelogenesis is faster and more organized than passive re-mineralization because it involves a protein scaffold← which catalyzes crystal formation. Furthermore, the lack of REE means that gingiva cannot form junctional epithelium← with dental implants←. Understanding how junctional epithelium forms in the first place improves our ability to trick oral mucosa cells into behaving more like junctional epithelium.
Lastly, it is interesting to consider why only two sets of teeth erupt, making us (and most mammals) diphyodonts
. The teeth of many other animals are exfoliated and replaced repeatedly throughout life (polyphyodonts
). Why don’t tooth buds recapitulate the exfoliation pattern of hair follicles? The answer is likely our oldest mamallian ancestors were tiny and did not live long. They lived long enough to benefit from replacing hair follicles seasonally (you dog owners understand). But multiple sets of teeth would not have been beneficial. So why two sets of teeth? For that, we must go back even futher in evolutionary time. The ancestors of the very first mammals developed one set of teeth for chewing their way out of a hard eggshell, then a second set for eating food. Apparenly, our ontogeny recapitulates that phylogeny. So why did we say most mammals? The teeth of elephants
and manatees
grow very differently from our own. Their teeth erupt from the distal end of the jaws and pushing older teeth mesially, not twice but repeatedly throughout their lifetime. Should we study elephants and manatees to see if we can repeat this in humans? That is up to you, but time is running out.
Summary of dental tissues
enamel | dentin | pulp | cementum | PDL | alveolar bone | |
---|---|---|---|---|---|---|
produced from | enamel organ | dental papilla | dental papilla | dental sac | dental sac | dental sac |
lineage | ectoderm | neuro-mesenchyme | neuro-mesenchyme | neuro-mesenchyme | neuro-mesenchyme | neuro-mesenchyme |
tissue type | epithelial | connective | connective | connective | connective | connective |
formative cells | ameloblasts | odontoblasts | fibroblasts | cementoblasts | fibroblasts | osteoblasts |
cells in mature tissue | (odontoblastic processes ) | odontoblasts
fibroblasts NMSCs |
cementocytes | fibroblasts
cemetoblasts osteoblasts PDLSCs |
osteocytes | |
resorptive cells | odontoclasts | cementoclasts | osteoclasts | |||
resorptive cell activity | exfoliation | exfoliation | throughout life, changes during eruption and exfoliation | |||
Mineral% | 96% | 70% | 50% | 66% | ||
Organic & water% | 1% protein
3% water |
20% protein
10% water |
38% protein
12% water |
21% protein
12% water |
||
major protein(s) | amelogenin
enamelin |
collagen | collagen
fibronectin |
collagen | collagen | collagen |
Forms during | embryonic | embryonic (crown)
post-natal (roots) |
embryonic (crown)
post-natal (roots) |
post-natal (roots) | tooth eruption | tooth eruption |
Vascularity | vascular | vascular | vascular |
Clinical considerations
Induction stage complications
Problems with the induction of tooth germ leads to the formation of too few or too many teeth.

“Incontinentia pigmenti presenting as hypodontia in a 3-year-old girl: a case report”
By Kitakawa et al, Journal of Medical Case Reports 2009, is licensed CC BY 2.0Anodontia
Failure during the induction stage results in missing teeth, or anodontia. This may include inadequate release of morphogens← by neuro-mesenchymal stem cells, mutations in the morphogen receptor proteins, or teratogens that interfere with activation of signal transduction cascades←. Partial anodontia (also called hypodontia), where one or a few teeth are missing, is most common. The most commonly missing teeth are permanent maxillary lateral incisors, 3rd molars, and mandibular 2nd pre-molars.
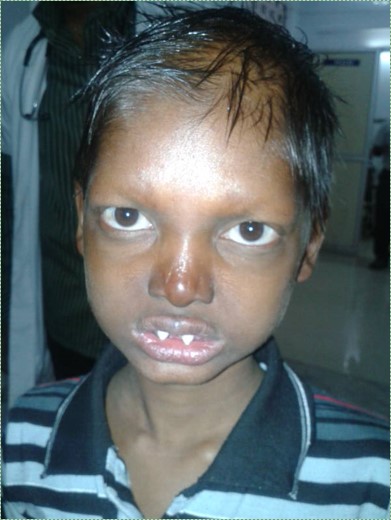
"Ectodermal dysplasia
"
, by Pratheeba227 – photographed at Govt Vellore Medical College, is licensed CC BY SA 3.0Ectodermal dysplasia (part 2 of 2)
Ectodermal dysplasia← is covered in chapter 6 where we discuss the induction of neural crest cells←. When signals from neural crest cells← are reduced, regions of ectoderm that are supposed to grow faster than others do not get the boost in growth they need. This includes hair follicles, finger and toenails, tooth buds, sweat glands and salivary glands. This may cause some or all of these structures to not develop at all (for teeth, anodontia), to develop in reduced number (for teeth, hypodontia), or reduced in size (for teeth, microdontia).
For those who cannot afford or are too young for dental implants←, complete or partial anodontia may be visible, as well as microdontia (Fig. 8.16). Children do not receive dental implants because implants do not grow in size to match a growing jawline. Multiple sets of dentures are an option (again, for those who can afford the treatment). Now is a good time to double-check you can distinguish between hypodontia and microdontia. If you haven’t had Latin or a good medical terminology class, remember a hypo-dermic needle goes lower than the dermis, while micro-biology is the study of small stuff.

"Fig. 5"
by Toby Hughes et al., J. Dental Anthropology
is licensed under CC BY 4.0Hyperdontia
Hyperdontia, the formation of extra (supernumerary) teeth, occurs when induction of tooth germ occurs where it shouldn’t. This is often a genetic condition. The most common extra teeth are between the central incisors (mesiodens), distal to the maxillary 3rd molar (4th molar, or distomolar) and the premolar region of either dental arch (perimolar).
Bud and cap stage complications
Problems in the bud stage of tooth development may lead to teeth that are too large (macrodontia) or too small (microdontia). This may affect all the teeth, some teeth, or a single tooth.

"Periapical radiography of superior central incisive permanent geminated"
by Katia Simone Alves dos Santos et al., Intl J Morphology
is licensed under CC BY 4.0Gemination
Tooth gemination (twinning, as in the constellation Gemini
) occurs when a single tooth germ is partially divided in two. This may occur if the growing tooth germ bumps into a small dense region in the dental arch. Under healthy conditions, teeth grow within loose mesenchyme← tissue, which later differentiates← into osseous tissue and calcifies. A geminated tooth, coming from a single tooth germ, is larger than average, but has a single pulp cavity, hence this patient has an instance of macrodontia (but not hypodontia).
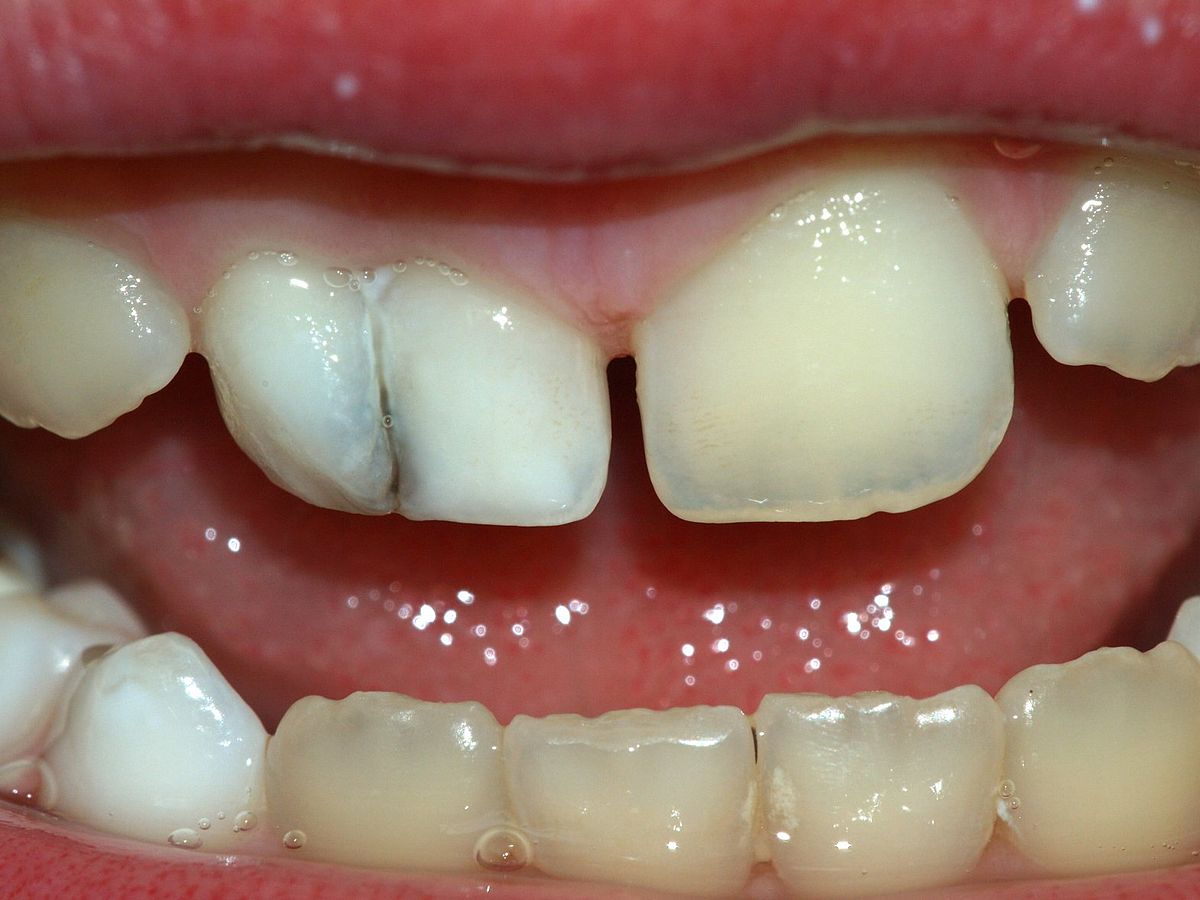
"milk.teeth.fusion"
by Sarefo
is licensed under CC BY-SA 3.0Fusion
Tooth fusion (joining) of two tooth germs into a single germ can also cause a larger-than-average tooth to develop. In this case, macrodontia is accompanied by hypodontia. The fused tooth has two separate pulp cavities. This occurs when induction of two tooth germs occurs close to one another, or when external pressure forces two tooth germs closer together. Tooth germs can fuse during early stages of tooth development because the tooth germ is soft epithelial and mesenchymal← tissues. Getting too close together during later stages, when enamel and dentin are calcified, leads to plain-old tooth crowding (malocclusion
).
It is worth a mention that the fusion of teeth involves two separate buds growing into one large structure when their secretions meet. Syndactyly, the fusion of toes or fingers, is not really a fusion but a lack of separation due to diminished or absent apoptosis← signals.

"Radiographic view of Teeth"
by Eduardo Borie E, et al, Intl J Morphology
is licensed under CC BY 4.0Dens in dente
Dens in dente (tooth within a tooth, or dens invaginitus) occurs when a small region of the enamel organ grows too fast and invaginates a second time, into the dental papilla, during the cap stage. This sub-region of the enamel organ continues to develop like a smaller version of the original enamel organ, creating what looks like a miniature tooth within the main tooth. This can complicate a root canal surgery, but otherwise only makes for interesting radiographs.
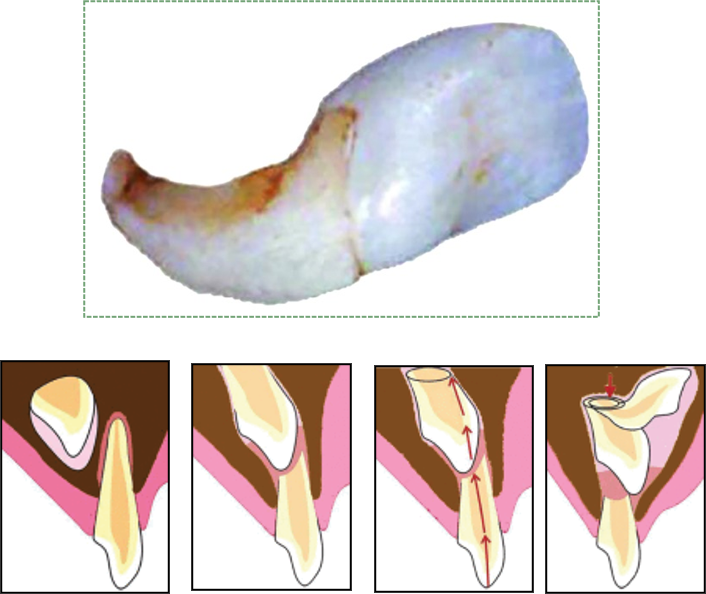
"Figs 1-5"
by Pawanjit Singh Walia, Intl J Clinical Ped Dentistry
is licensed under CC BY 3.0Dilaceration
Dilaceration, or a bend in the shape of a tooth, occurs when there is an impediment to the growth of HERS. A delay in tooth eruption can cause dilaceration. This can occur because the crowns develop and calcify first, and the roots develop later. If a developing tooth bumps into calcifying osseous tissue and is forced to change its direction of growth, the older calcified part of the tooth (the crown) will have grown at a different angle from the newer, softer root(s). Alternatively, trauma to a deciduous tooth can be transferred to the deeper succedaneous tooth, causing dilaceration of the succedaneous tooth (Fig 8.21).
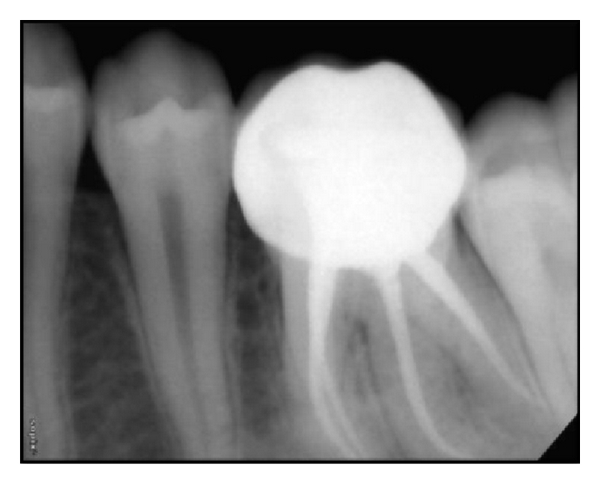
"Postoperative IOPA of 36 and 46."
by Muktishree Mahendra et al, Case Reports in Dentistry
is licensed under CC BY 3.0Supernumerary roots
Teeth may develop extra, or supernumerary roots. As with dens in dente, this may complicate root canal surgery, but otherwise has limited clinical significance. When the shape of the tooth is altered but no pathology results, clinicians may note there is an alteration to the morphology of the tooth.
Eruption complications

"Own work"
By Daniel Schwen – is licensed under CC BY-SA 4.0Teething
During tooth eruption, several tissues undergo significant remodeling, including the REE, oral epithelium and bone tissue←. This involves removal of ECM by the secretion of digestive enzymes, and removal of cells by apoptosis←. However, enzymes aren’t smart enough to remove only ECM, they tend to kill cells as well. For instance, when the enamel organ secretes matrix metaloproteinases to clear a path for the erupting tooth, this triggers inflammation as plasma membrane proteins are destroyed along with ECM, leading to cell death. Inflammation can cause discomfort, tenderness or edema in the affected area.

"Impacted Wisdom Tooth aka Lower Right Third Molar 48 RVG IOPA Xray"
by Nizil Shah
is licensed under CC BY-SA 4.0Primary failure of eruption
Primary failure of eruption (PFE) is the partial or complete failure of a tooth to erupt (otherwise known as an impacted tooth
) despite a healthy eruption pathway. While there are likely many causes of PFE, one major heritable cause is a mutation that disrupts parathyroid hormone signaling
from the tooth germ to alveolar bone tissue. Parathyroid hormone activates osteoclasts and inhibits osteoblasts, therefore inhibiting parathyroid hormone signals leads to increased bone deposition. This leads to ankylosis of the tooth prior to eruption. It also makes tooth extraction difficult or impossible, especially as time progresses. Impacted teeth may cause inflammation and pain, or may not be detected until an x-ray is performed. The 3rd molars are the most commonly impacted teeth. Complications include crowding of neighboring teeth, infection, malocclusion
, and– rarely– neoplasia
, making extraction an important option.
Mechanical failure of eruption
A tooth may also become impacted after erupting partially because of ankylosis of the tooth, which is termed mechanical failure of eruption (MFE— it seems odd that it is not secondary). In contrast to PFE, there is a physical barrier to the eruption pathway. Absence of the PDL indicates ankylosis and can indicate MFE. With MFE, orthodontic or other therapies may allow the impacted tooth to erupt eliminating the need for extraction and replacement.

"Jaw cyst"
By: Coronation Dental Specialty is licensed under CC BY 3.0Dentigerous cyst
An impacted tooth can produce a cyst around the crown, called a dentigerous cyst. As the motionless tooth attempts to digest its way through oral epithelium, fluid accumulates between the crown and REE. The cyst may continue to grow in size, causing pain and trauma to the jaw bone (usually the mandible). Extraction of the impacted tooth will solve the issue. Orthodontic facilitation of tooth eruption may be an option as well. Rarely, the REE in a dentigerous cyst develops into a benign tumor known as an ameloblastoma
. The main risk of this cancer is that similar to that of the cyst—it can cause pressure which may cause bones to break or to grow malformed. The reason this tumor is rare is that the REE cells are terminally differentiated (non-mitotic). Furthermore, because these epithelial cells are anchored to one another by desmosomes←, this type of tumor rarely metastasizes.

"Own work"
by West Exchange is licensed under CC BY 3.0Bohn’s nodules and Epstein pearls
Bohn’s nodules are masses of keratin trapped in the gingiva. They may be referred to as epithelial rests of Serres in older texts, and are also called Epstein pearls when they are located within oral mucosa. Bohn’s nodules are produced by the REE, while Epstein pearls are produced by oral epithelium. Furthermore, remnants of minor salivary glands can be called Bohn’ nodules as well, despite lacking keratin. Their presence along the alveolar ridge may cause parents to mistake them for erupting teeth. They occur more commonly along the maxillary ridge. Bohn’s nodules and Epstein pearls are transient—they go away on their own, usually within 3 months, and produce few if any disturbances.
Lastly, if you are interested in the history of embryology and long-dead European men, we offer the following. Antoine Étienne Serres collaborated with the embryologist Johann Friedrich Meckel, who named the cartilage in the mandibular arch that develops into the mandible (Meckel’s cartilage). Together, they attempted to unify embryology and the lineage of different species (later called evolution by heritable selection by Charles Darwin
and the less-well-known work of Alfred Russel Wallace
). Serres’ and Meckel’s theory was developed further by Ernst Haeckel, who coined the memorable phrase “ontogeny recapitulates phylogeny,” which we have quoted several times in this text.
< Chapter 7 * navigation * Chapter 9 >
Chapter review questions
The region where the ectoderm and endoderm come into direct contact with each other constitutes a thin membrane, which forms a septum between the primitive mouth and pharynx.
Periodontal Ligament, a group of specialized connective tissue fibers that attach a tooth to alveolar bone.
Epithelium on the inner wall of the gingiva that attaches to connective tissue of the lamina propria on the basolateral side and to the surface of the tooth on the apical side.
Small bumps that give rise to bigger structures such as hair follicles and teeth.
The embryonic process in which one group of cells directs the development of another group of cells.
The mucous membrane lining the inside of the mouth. It is a stratified squamous epithelium, named the oral epithelium, and an underlying areolar connective tissue named the lamina propria.
The process of cell division, where one cell divides into two identical clones (daughter cells).
A substance whose non-uniform distribution governs the pattern of tissue development and pattern formation.
A temporary group of cells that arise from the embryonic ectoderm, and in turn give rise to a diverse cell lineage—including melanocytes, cranio-facial cartilage and bone, teeth and periodontal tissue, smooth muscle, peripheral and enteric neurons and glia.
Hematoxylin and Eosin stain, typically turns proteins pink and DNA purple.
The developmental history of a differentiated cell traced back to the embryonic cell from which it arises.
he most exterior of the three primary germ layers formed in the gastrula, it gives rise to the epithelial tissue covering the body and the CNS.
Mesenchyme tissue that contains neural crest cell derivatives.
A hard connective tissue which is mostly ECM, including collagen fibers and a calcium-phosphate crystal.
Extracellular matrix material involved in the repair of injured and missing tissues, allows stem cells to migrate into the injured area. It is usually replaced during regeneration.
Reorganization or renovation of existing tissues, either physiological or pathological. The process can either change the characteristics of a tissue such as in blood vessel remodeling, or result in the dynamic equilibrium of a tissue such as in bone remodeling.
After partial loss of as tissue, based on the remaining part, the tissue grows the same structure and function as the lost part.
An embryonic tissue composed of undifferentiated mesenchymal stem cells and mucous ground substance.
The middle of the three embryonic layers that develop during gastrulaton, mesoderm becomes most of the body's muscle and connective tissues.
The three layers of cells that arise from gastrulation: endoderm, mesoderm and ectoderm.
Shared equally by two parties.
The process by which animals and plants grow and change.
Formation and eruption of the teeth.
The folding process in vertebrate embryos, which includes the transformation of ectoderm into the neural tube.
Inward folding of an epithelium caused by interstitial growth.
A mass of tissue having the potentiality of differentiating into a tooth.
A band of epithelial tissue seen in histologic that is is the first evidence of tooth development and begins (in humans) at the sixth week of development.
The first branchial arch of the vertebrate embryo which in humans develops into the lower lip, mandible, masticatory muscles, and anterior tongue.
A process that grows off the mandibular arch on each side and forms the lateral part of the upper lip, cheek, and upper jaw except the pre-maxilla.
The embryonic precursor to the central nervous system.
A process by which epithelial cells lose polarity and cell-cell adhesion, and gain migratory and invasive properties to become mesenchymal stem cells, this normally occurs during embryonic development and wound healing.
Enzymes that degrade all kinds of extracellular matrix proteins, or process a number of bioactive molecules. They play a major role in cell proliferation, migration, differentiation, angiogenesis, and apoptosis.
A process in tooth development in which the teeth enter the mouth and become visible.
When two or more cells, tissues or organs join to become one.
A subset of mesenchymal stem cells derived from neural crest cells rather than mesoderm, or mesoderm-derived mesenchymal stem cells induced by neural crest morphogens to adopt a more neural fate.
A polarity axis that organizes cells in the plane (side-to-side) of the tissue.
Fibroblast Growth Factors are a family of morphogens involved in a wide variety of processes, including important roles in development and tissue regeneration, especially connective tissues.
Bone Morphogenetic Proteins are a group of signaling molecules, initially discovered for their ability to induce bone formation, now known to play crucial roles in all organ systems.
When one cell begins to look different from another. This process involves limiting cell fate by altering gene transcription to become more specialized.
The process that results in an increase of the number of cells, and is defined by the balance between cell divisions and cell loss through cell death or differentiation.
The first visible stage of odontogenesis, without a clear arrangement of cells.
The type or types of cell(s) a stem cell can possibly differentiate into in the future, determined by which genes are methylated and stored around histones, or free to be transcribed.
The areolar connective tissue layer of the oral mucosa (or hollow organ), homolgous to the papillary layer of the dermis.
An adjective used to describe a gelatinous, slimy substance such as loose ground substance or mucus.
Gel-like substances in the ECM, composed of water held in place by large molecules (proteins, glycoproteins and glycosaminoglycans).
A condensation of ecto-mesenchymal cells seen in histologic sections of a developing tooth.
An early stage of tooth development where ectodermal cells grow around the dental papilla to resemble a hat, and the first signs of cell arrangement occur.
The ectodermal cells that lie above the dental papillae in a cap stage tooth bud.
Neuro-mesenchymal cells and fibres surrounding the enamel organ and dental papilla of a developing tooth.
An aggregation of cells that eventually forms a tooth, composed of the enamel organ, dental papilla and dental sac.
To state again, or to repeat.
The stage of early tooth development where the dental organ differentiates into 4 distinct cell types 9IEE, OEE, stellate reticulum and stratum intermedium), followed by differentiation of odontoblasts and ameloblasts.
Inner Enamel Epithelium: a layer of columnar cells located next to the dental papilla, these pre-ameloblast cells will differentiate into Ameloblasts which are responsible for secretion of enamel during tooth development.
Outer Enamel Epithelium: a layer of cuboidal cells located on the periphery of the enamel organ in a developing tooth.
Cells present only during the embryonic period that deposit tooth enamel.
A stratified layer of cells situated between the IEE and the stellate reticulum.
A group of star-shaped cells located in the center of the enamel organ of a developing tooth, they synthesize glycosaminoglycans.
A cell of neural crest origin that is part of the outer surface of the dental pulp, and whose biological function is the formation of dentin.
A thin sheet of extracellular matrix between epithelial tissues and the underlying connective tissue of the lamina propria or dermis.
Extra-Cellular Matrix: Materials in the body, but outside of cells. A network of macromolecules, such as fibers, enzymes, and glycoproteins, that provide structural and biochemical support to surrounding cells.
An intermediate cell type, differentiate from IEE cells, capable of inducing the differentiation of odontoblasts, which in turn induce pre-ameloblasts to differentiate into ameloblasts,.
An epithelium composed of one layer of square cells
Epithelia that have one layer of tall cells
Newly formed dentin before calcification and maturation.
The formation of dentin.
The formation of enamel.
Arm-like extension of an odontoblast found within dentinal tubules.
A histologic landmark identified on an ameloblast.
Sometimes referred to as the maturation stage or the late bell stage, characterized by the calcification of enamel and dentin in the crown region of a developing tooth.
The increase in diameter by the addition of tissue at the surface,
The side of a cell or tissue oriented away from the lumen or external surface.
The location on an enamel organ in a developing tooth where the outer enamel epithelium and the inner enamel epithelium join, fated to become Hertwig's Epithelial Root Sheath (HERS).
Hertwig's Epithelial Root Sheath: A proliferation of epithelial cells located at the cervical loop of the enamel organ in a developing tooth which initiates the formation of root dentin.
Programmed cell death
Clusters of epithelial cells found within the PDL, remnants of Hertwig's Epithelial Root Sheath (HERS).
Differentiated cell that deposits cementum matrix.
Cementum covering the cervical portion of the tooth root, important for attachment of the periodontal ligament (PDL) to the root surface.
Cementum containing cells and collagen fibers anchoring the tooth to alveolar bone.
Terminally differentiated cell found within mature cementum lacunae.
Lakes, or spaces within cartilage of bone connective tissue where cells reside.
The main structural protein in the ECM of connective tissues
The biological process that causes a cell, tissue or organism to develop its shape.
The basic connective tissue cell type capable of secreting ECM components, including fibers and ground substance proteins.
A differentiated connective tissue cell that secretes bone ECM, including collagen, calcium and phosphate.
The part of the jaws that holds the teeth.
Tooth movement in the occlusal direction as the tooth erupts from its osseous crypt.
A cell derived from bone marrow stem cells capable of demineralizing bone tissue by the secretion of acids and enzymes, releasing calcium into the blood.
Osteoblasts and osteoclasts working together to remove old bone tissue and replace it with new bone tissue, necessary for the maintenance of healthy bones.
A rare congenital malformation that affects the collarbones, skull and teeth.
The pressure exerted by liquid.
Receptor activator of nuclear factor kappa-Β ligand, a pro-apoptosis gene expressed in many tissues.
Signaling molecules secreted directly into the blood which travel throughout the body.
A terminally differentiated epithelial cell capable of synthesizing large amounts of the protein keratin within its cytoplasm.
Movement of the gingiva apically or away from the crown of the tooth to the level of the CEJ after the tooth has erupted completely.
Cemento-Enamel Junction, found in the cervical region of the tooth.
The dense irregular connective tissue layer found below the oral mucosa (or mucosa of a hollow organ), homologous to the reticular layer of the dermis.
Inflammation of gingival tissue
Reduced Enamel Epithelium: the OEE and ameloblasts found on the surface of the crown prior to tooth eruption.
The stratified squamous epithelium of the oral mucosa.
Trans-membrane structure specialized for cell-to-cell adhesion.
Cell Adhesion Molecules: proteins located on the cell surface involved in binding with other cells or with the extracellular matrix.
Half of a desmosome, specialized for cell-to-ECM adhesion.
The top (or apex) side of a cell or tissue, usually facing the lumen.
A thin membrane of remaining REE tissue that covers the tooth once it has erupted.
An artificial tooth root that is surgically placed into bone tissue.
A general term for peeling or shedding, in humans it refers to the loss of dead epithelial cells or structures (hair, teeth).
An infolding of the epidermis that extends deep into the dermis, responsible for producing a hair.
The development of an organism or anatomical or behavioral feature from the earliest stage to maturity.
The history of the evolution of a species or group, especially in reference to lines of descent and relationships among broad groups of organisms.
absence of primary or permanent teeth.
A cell-surface, trans-membrane or cytoplasmic protein that binds to (receives) a signaling molecule and transmit the signal further.
Agents or factors which cause malformation of an embryo.
When a chemical or physical signal is transmitted through a cell as a series of molecular events, most commonly protein phosphorylation catalyzed by protein kinases, which ultimately results in a cellular response.
Partial anodontia, or the absence of some teeth.
A group of disorders in which two or more of the ectodermally derived structures — the skin, sweat glands, hair, nails, teeth and mucous membranes — develop abnormally.
A condition in which one or more teeth appear smaller than normal
the condition of having teeth in addition to the regular number of teeth.
A supernumerary tooth present between the two central incisors.
A supernumerary tooth which is located distal to third molars
A supernumerary tooth in front of the molar teeth.
A condition in which one or more teeth grow to be a larger size than normal.
Adental phenomenon that appears to be two teeth developed from one.
The union of two adjacent teeth at the crown level (enamel and dentin).
A rare dental malformation found in teeth where there is an infolding of enamel into dentin.
An abnormal bend in the root or crown of a tooth.
Extra roots on a tooth.
The shape (or form) of.
The border of every cell, made of a phospholipid bilayer and proteins.
Swelling caused by excess fluid trapped within tissues.
Partial or complete failure of a tooth to erupt despite a healthy eruption pathway.
Abnormal stiffening and immobility of a joint (including tooth-to-alveolar bone)
A type of failure of eruption takes place when the affected tooth is ankylosed to the bone around it.
A fluid filled pocket or sac around an impacted tooth.
When a cell has finished its last possible differentiation step and lost the ability to undergo mitosis.
Keratin cysts derived from remnants of odontogenic epithelium over the dental lamina, or remnants of minor salivary glands. They occur on the alveolar ridge, more commonly on the maxillary than mandibular.
One of a family of tough fibrous structural proteins, the key material making up hair, nails, calluses, and the outer layer of skin.
A white or yellow bump on the oral mucosa which are equivalent to Bohn's Nodules.
Small salivary glands located throughout the oral cavity that generally get no name.
Apiece of cartilage from which the mandibles (lower jaws) of vertebrates evolved, originally the lower of two cartilages which supported the first branchial arch in early fish.