9 Enamel development
- Overview
- Amelogenesis
- Initiation
- Apposition
- Mineralization
- Clinical considerations

"The sequential and reciprocal regulatory signaling between epithelium (red) and mesenchyme (blue)"
by Thesleff, I. and Tummers, M, Stembook.org
, is licensed under CC BY 3.0Overview
Sola dosis facit venenum (the dose makes the poison) — Paracelcus
Even though dentin formation begins before enamel, we begin with enamel formation. This way, the chapter on dentin formation can remain close to pulp, which is important because they share the same lineage from neuro-mesenchyme. Enamel is produced by cells derived from the ectoderm. This leads to key molecular differences between the ECM of enamel versus the ECM of dentin, cementum and bone tissue.
One major concept we focus on is that ameloblasts and odontoblasts undergo reciprocal signaling, meaning you can’t get odontoblasts without ameloblast signals, and you can’t get ameloblasts without odontoblast signals. It takes a team effort. In this chapter, we mention three morphogens← (ones we’ve covered before), and two transcription factors. Hopefully, Fig. 9.1 illustrates that the process is more complex. Don’t memorize the morphogens unless your instructor indicates they are on your exams. The important concept is that when we get to chapter 10 and discuss how dentin undergoes regeneration, we should question how it is similar to embryonic development, and how it is different as well.
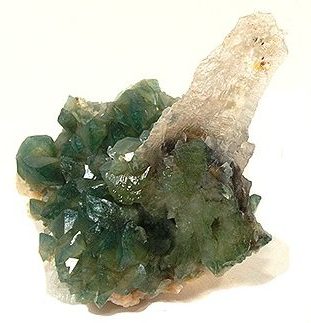
One reason to study embryology is to learn about how tissues are made in the first place. When we do, we often learn how to repair tissues better. For bone and oral mucosa, learning how stem cells← differentiate← into adult cell types has led to advances in bone grafting, guided tissue regeneration← and bio-active membranes. Could we one day re-grow enamel using stem cells? In this chapter, think about why creating new ameloblasts might not be as useful to us as new osteoblasts or fibroblasts (hint: location, location, location). Then, focus on how enamel matrix is formed. Secrete some proteins and minerals and turns into teeth and bones, right? Fig. 9.2 shows fluorapatite crystals. A mouth full of those would look impressive, but wouldn’t be very functional. Calcium and phosphate react to form a powder, you can probably find a bottle of it in your school’s chemistry lab. To get these chemicals to react in a way that creates human teeth requires more than chemistry. So perhaps the biggest concept in this chapter is how ameloblasts create calcium hydroxyapatite crystals in the shape and density of a tooth, rather than in the shape of Fig. 9.2. To understand tooth morphology, you must understand how its ECM is created.
In this chapter and the next, we discuss Tomes' process, Tomes' granular layer and Tomes’ fibers (we use the name odontoblastic process instead). If you wish, take a look at:
Tomes' collection of teeth, skulls and dental instruments
- type “tomes, sir john” into the search tool
- the Royal College of Surgeons of London (just don’t ask where he acquired these, apparently)
Amelogenesis
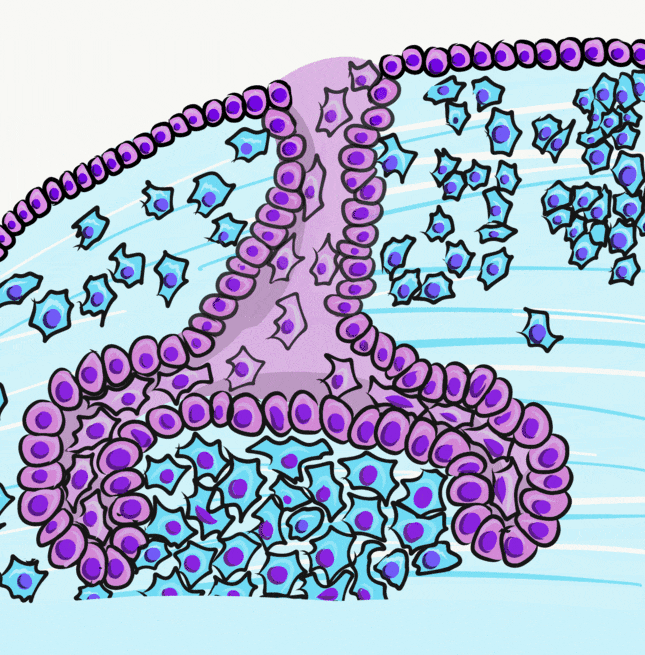
Induction (or initiation)
This paragraph should be review. Amelogenesis starts at the bell stage of tooth development. By this stage, ectodermal cells have invaginated to produce two layers of simple cuboidal cells← called the inner enamel epithelium (IEE) and outer enamel epithelium (OEE). Between the IEE and OEE, more epithelial cells are scattered, namely the stratum intermedium and stellate reticulum cells. Collectively, these cells are known as the enamel organ.
Now let us add to what we learned in the previous chapter. The enamel organ grows around a cluster of neuro-mesenchymal stem cells known as the dental papilla. Initially, a basement membrane separates the two. The first visible sign of differentiation← occurs when the cuboidal IEE cells next to the dental papilla elongate, becoming more columnar in shape. Their nuclei move to the apical side of the cell, while organelles needed for secretion accumulate on the basal side. We call these elongated cells pre-ameloblasts. It is not certain what triggers this initial change in morphology, evidence suggests a member of the Wnt family of morphogens← activates planar cell polarity← signals. The ability to undergo mitosis← halts, meaning no new pre-ameloblasts form after the bell stage. The disappearance of the basement membrane allows these cells to move more easily, due to the loss of hemi-desmosome attachments.
Pre-ameloblasts secrete a number of short-range morphogens←, including a member of the BMP family (Fig. 9.3). This induces neighboring neuro-mesenchymal stem cells of the dental papilla to differentiate into odontoblasts and begin secretion of pre-dentin. This puts pre-ameloblasts in direct contact with pre-dentin. Integrins on pre-ameloblasts contact a form of collagen← found in pre-dentin (collagen type I), as opposed to the type in the basement membrane (collagen type IV). In addition, newly formed odontoblasts secrete several morphogens, including BMPs. The important concept is that half of the signal that induces the differentiation of ameloblasts comes from the pre-dentin side.
The second half of that signal comes from the opposite direction, the stellate reticulum. One such signal produced by stellate reticulum cells includes a member of the FGF family. This leads to the activation of transcription factors in the pre-ameloblasts, which up-regulate the expression of genes involved in the secretion of enamel. Transcription factors involved in amelogenesis include MSX-2, a homeobox← gene, and RUNX2, a transcription factor also involved in the differentiation of osteoblasts. Visible under the microscope, pre-ameloblasts change shape again, becoming shorter, and develop a bump on the basal side known as Tomes’ process. A summary of some molecules involved is in Table 9.1. The names are less important than the general order of events: from morphogen← to receptor to transcription factor to gene expression.
Morphogens |
Receptors | Transcription factors | Genes up-regulated |
---|---|---|---|
Collagen (pre-dentin) BMP (odontoblasts) |
Integrins BMP-R |
MSX-2 DLX1 RUNX2 |
Enamelin Amelogenin Matrix Metalloproteinase |
FGF (stellate reticulum) | FGF-R |

18036
” is in the Public Domain, CC0 / croppedApposition
After changing morphology, ameloblasts secrete enamel matrix from Tomes’ process. This ECM is similar to the ECM of bone tissue←, but with important differences. Like bone matrix, enamel matrix is first secreted in a softer form (pre-enamel), consisting mostly of proteins and water, and it mineralizes later. Enamel matrix contains Calcium-Phosphate crystals and organic components. Enamel, after it matures, has a higher mineral percentage, making it the hardest substance in the human body. Unlike bone tissue, however, enamel matrix has very little or no collagen←. Instead, ameloblasts secrete other proteins known as amelogenins and enamelins. These proteins act as nucleation centers
which help induce Calcium and Phosphate to form crystals. We can think of these proteins as scaffolds← for non-living chemicals rather than cells. The important fact to remember here is that collagen is a protein commonly made by fibroblasts (and other cells derived from mesenchyme), which most often differentiate← from the mesoderm. Ameloblast lineage, on the other hand, is from the ectoderm. Presumably the connective tissue genes like collagen are methylated← and packed away around histones, and are not available for transcription.
The shape of the enamel organ in the bell stage determines the shape of the crown. No new ameloblasts form because they lose the ability to undergo mitosis← once they differentiate← into pre-ameloblasts. Furthermore, no stem cells← can contact both pre-dentin and stellate reticulum once thick layers of enamel and (mature) dentin push the two apart. Therefore, no new ameloblasts form by differentiation, either. Because of how the pre-ameloblasts line up in the bell stage, mostly pointing towards the oral cavity, enamel is thicker in the masticatory surfaces and thinner in the cervical areas.
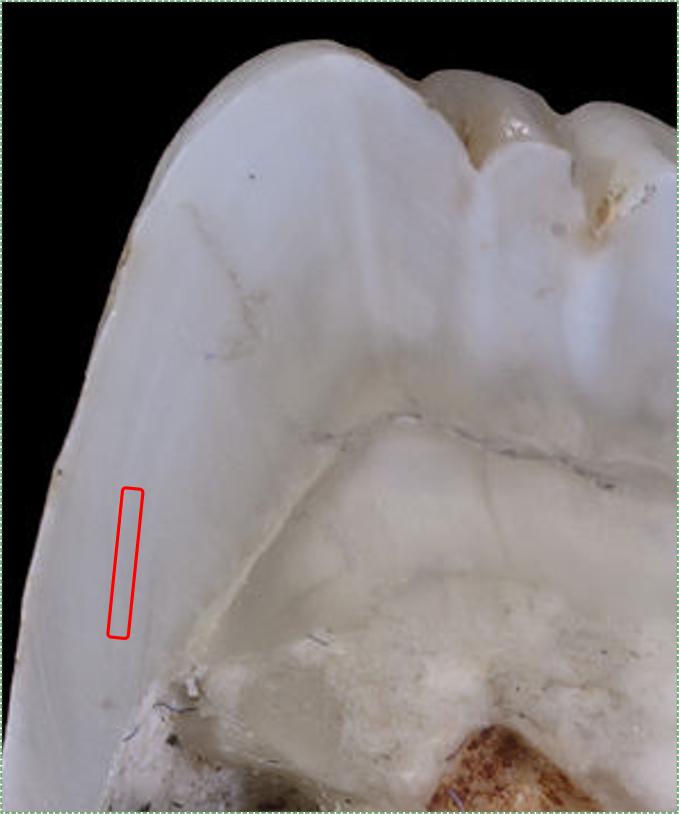
"Tooth of Paranthropus robustus SKX 21841 from Swartkrans"
by Didier Descouens
is licensed under CC BY 3.0 / red box addedEnamel is secreted by ameloblasts from Tomes’ process, laying down new layers of pre-enamel over older layers, pushing the ameloblasts further away from dentin. Ameloblasts, like many human cells, do not work at a uniform speed. About every 4-12 days there is a change in the enamel deposition rate. As a result of deposition of enamel speeding up and slowing down, visible bands of lighter and darker enamel (less dense and more dense) are visible when viewed in cross section. These visible bands are known as Lines of Retzius (or striae of Retzius, Fig. 9.5). One such band, the neonatal line, is particularly dark because the ameloblasts rested during the day we were born. After birth, the edges of the Lines of Retzius may be visible on the surface of teeth, especially the lingual surface of anterior teeth, in which case they are called perikymata (in older texts, the imbrication lines of Pickerill). Over time, chemical and physical abrasion wear perikymata away.
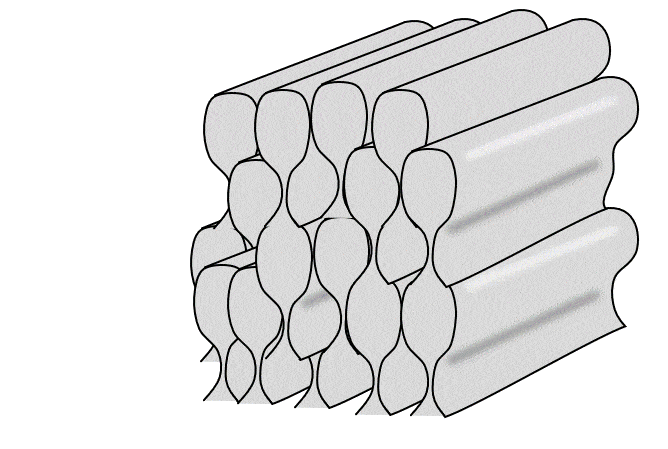
Together in a line, all of the ameloblasts produce horizontal layers of enamel, new on top of old. But roughly one ameloblast produces a single enamel rod (or prism) in a perpendicular direction (it takes 4 ameloblasts to make one enamel rod, but each ameloblast works on 4 different rods). Because the rods are rounded (almost hexagonal), and not square, there are gaps between them. These gaps aren’t empty, they are filled with a slightly different form of enamel, called inter-rod enamel (while the enamel within rods is called rod enamel).

18036
” is in the Public Domain, CC0 / croppedIf you look closely to Fig. 9.6, you might notice Hunter-Schreger bands (HSB), which are lines running perpendicular to the Lines of Retzius and the DEJ. Each line represents the contributions of a small group of ameloblasts. If it’s a little hard to see, it is because each Hunter-Schreger band is only 1/10th of a millimeter thick. What you are looking for is a pattern similar to the fore edge of a textbook (all the pages as a group). Ameloblasts do not work in straight lines, the bands curve slightly– like the pages of that $375 textbook you dropped in water and can’t sell back to the bookstore. Furthermore, different regions have different curve angles and patterns. These curves aren’t accidental, they increase the tensile strength of enamel. If you have ever chopped wood, you know it is easy to do if you land the axe along the grain. Hit at an angle, and it can hurt you instead. The curving of Hunter-Schreger bands ensures there is no single grain along which a tooth can easily shear. Cracks develop in enamel, but cracks usually stop part-way through. The planar cell polarity← signals that guide rod curvature have been identified (antagonists of Wnt morphogens
). The end result is that enamel, despite having the brittleness of glass
, is the most resilient tissue in the human body. Dentin has similar curves (plural) which, unlike enamel, get specific names. As a fun side-note, or of relevance to those planning on pursuing a career as a veterinary dental hygienist
at your local zoo, the Hunter-Schreger bands of hyenas occurs in a zig-zag pattern, increasing the tensile strength of their enamel even more, allowing them to chew through bones.
Maturation
Enamel is first secreted as a protein-rich gooey substance called pre-enamel. Two major organic components of enamel are the proteins amelogenin and enamelin. Ameloblasts also secrete a digestive enzyme. This enzyme, a matrix metalloproteinase, digests collagen←, making it easier for ameloblasts to migrate though the ground substance of overlying mesenchyme←. This is necessary because as ameloblasts deposit pre-enamel from Tomes’ process, it pushes the cells outwards. If you recall, matrix metalloproteinases are also involved in the proper migration of tissues during formation of the palate as well as in tooth eruption←. Matrix metalloproteinases serve a second role by processing enamel proteins made by the ameloblasts after they are secreted into the ECM. The enzymes modify (not digest) amelogenins and enamelins, improving their ability to catalyze the crystallization of calcium and phosphate. This creates a delay between the secretion of pre-enamel and its mineralization. Without a delay, ameloblasts could become trapped by their own ECM, halting amelogenesis.
Unlike dentin and cementum, enamel undergoes a significant amount of remodeling after mineralization. Once pre-enamel has been laid down and mineralizes, ameloblasts switch tasks and cause the hard enamel to mature into even harder enamel. Most of the proteins are removed, replaced by fluids which crystallize. Amelogenin and enamelin are removed by endocytosis and by secretion of protein-digesting enzymes. These enzymes include the matrix metalloproteinase whose first cuts to amelogenin and enamelin improved their function, but more cutting breaks them down. Removal of protein increases the relative mineral content of enamel, increasing its hardness compared to bone, dentin and cementum. We can therefore think of the protein secreted by ameloblasts as a scaffold← that ensures enamel is made quickly, at about 4 μM per day. In contrast, fluoride-based re-mineralization of tooth enamel is not measured using a small ruler, suggesting the rate of re-mineralization is too slow to see under the microscope.
A number of different electrolytes and molecules are incorporated into enamel in small amounts during the maturation stage. One important electrolyte is fluoride (F–). Fluoride ions are negatively charged, and incorporate into calcium hydroxyapatite crystals by replacing a small number of hydroxide (OH–) ions. This has important clinical effects. First, a small amount of fluoride increases the strength of calcium hydroxyapatite crystals. Secondly fluoride ions found in either saliva or in enamel neutralize acids released by bacteria, reducing enamel demineralization and preventing caries. Excessive fluoride levels, however, affect the activity of ameloblasts during the maturation stage of amelogenesis, reducing their removal of protein scaffolds←. This may create a visual change in the form of white lesions on the surface of teeth. These lesions are where the enamel has a higher protein and lower crystalline content, known as dental fluorosis. As with any chemical, the dose makes the poison
. Topical application, from toothpaste or gels, allows fluoride to incorporate into the surface layers of enamel, which has considerable benefits. The greatest potential benefit is when fluoride exposure occurs during the apposition and maturation stages of amelogenesis, early in embryonic development.
Clinical considerations
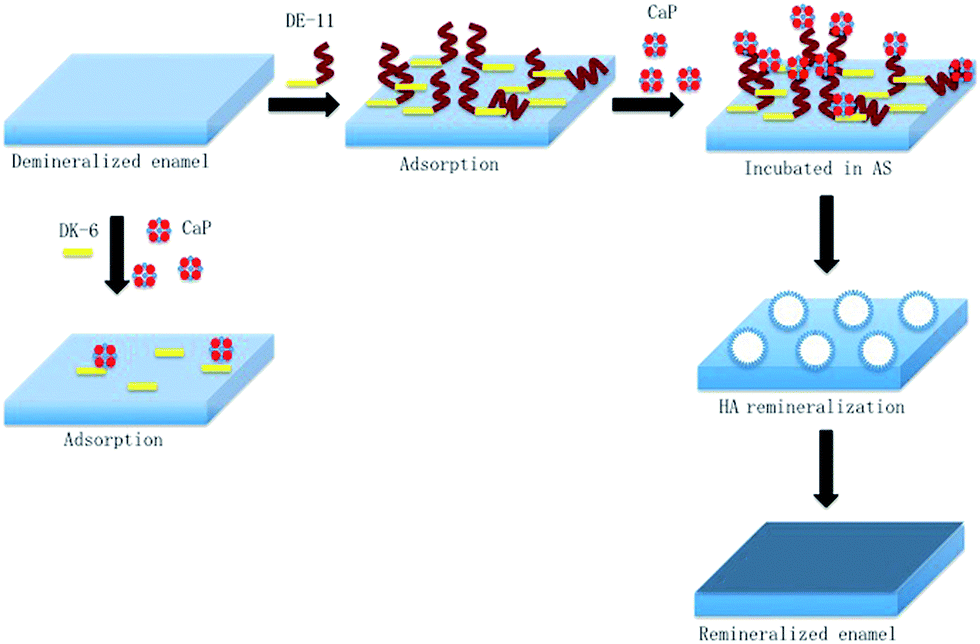
Schematic of the adsorption of DE-11 on the demineralized enamel surface for remineralization restoration
“ by Wang, K. et al is licensed under CC BY-NC 4.0Enamel re-mineralization
Certain chemicals help induce calcium and phosphate to react and add to the crystalline matrix of enamel. The most common is fluoride. In addition to its role in neutralizing acids, Ffuoride nucleates calcium hydroxyapatite crystals (see also Fig. 9.2). Topical gels and toothpastes that promote re-mineralization frequently contain Fluroride plus calcium and phosphate in forms that dissolve in saliva. Other agents are added to help fluoride to stick to the surface of enamel. After all, promoting the formation of calcium-phosphate powder in saliva won’t help teeth. One such agent is casein-phosphopeptide
. Casein is a protein found in milk. Its stickiness makes it useful in cheese-making and glue
(including the original formula of Elmer’s glue).
Increased understanding of how protein scaffolds← facilitate the growth of mature tissues has allowed researchers and companies to develop better tools for assisting in enamel re-mineralization.
The goal is to mimic the ability of amelogenin and enamelin proteins to nucleate calcium hydroxyapatite crystals from a solution containing calcium and phosphate. These products do not need to contain amelogenins, enamelins, or even proteins. For instance, xylitol
and certain glass compounds nucleate calcium hydroxyapatite crystals. Xylitol, a sugar alcohol extracted from birch trees, has the dual benefit of activating sweet taste buds. It can be used to replace simple sugars in foods, removing substrates which harmful oral bacteria use for energy when secreting enamel-dissolving acids (just don’t let your dog eat any).
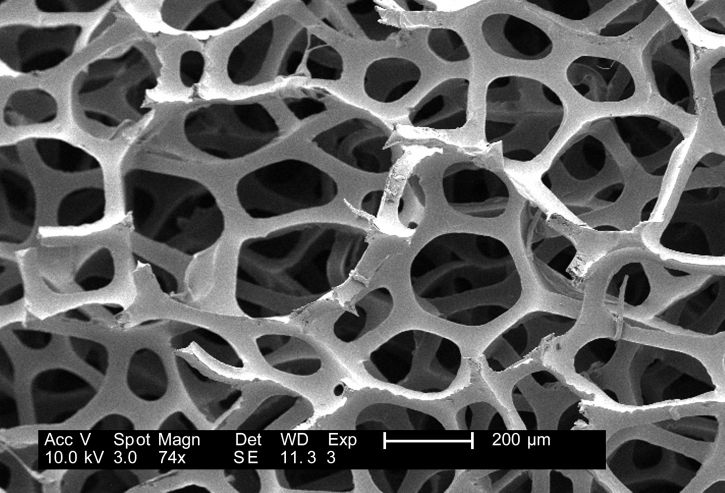
"This work"
by Janice Carr, USCDCP is in the Public Domain, CC0Surface etching
Because rod enamel is slightly different from inter-rod enamel, applying an acid to the crown surface wears the two at different rates—a process called surface etching. This creates an uneven surface, providing more surface area to create a stronger bond between the tooth and a surface sealant (think about how dermal papillae← and rete pegs← interdigit with one another for a stronger connection). Treatment with a weak acid also removes surface debris which interferes with bonding of an adhesive, known as a smear layer.
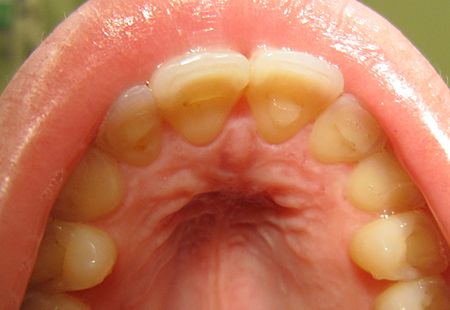
Erosion
Prolonged exposure to a weakly acidic environment, or a shorter exposure to a highly acidic environment, can cause erosion of tooth enamel. Positively charged H+ ions of an acid react with negatively charges OH– and OPO33- ions of calcium hydroxyapatite crystals, dissolving them. Bulimia and acid reflux bring highly acidic hydrochloric acid of the stomach in contact with teeth, causing erosion. Xerostomia, caused by the abuse of drugs such as methamphetamines, the use of certain medications, chemotherapy, or that associated with old age, reduces the protective buffering ability of saliva to protect tooth enamel. Acidic foods or sugary foods may also contribute to enamel erosion. The simple sugars in many foods are nutrients oral bacteria metabolize and use to produce energy, which they then expend to create and secrete enamel-eroding acids.

"Impacted wisdom teeth caries"
by Coronation Dental Specialty Group
is licensed under CC BY-SA 3.0Decalcification (a dental caries, or tooth decay) can be detrected earlyduring a regular examination. Early signs of decalcification include the appearance of a white lesion on the surface of enamel, which may be softer to the touch, and appear more radiolucent on a dental radiograph. Treatment often involves removal of the carious tissue followed by replacement with a bio-compatible material.

"Own work
” By Ebd2015 is licensed under CC BY 3.0Abfraction
When non-carious tooth material is lost by force, it is called abfraction. Abfraction frequently occur along the DEJ. Chewing causes flexion forces at the cervical region of the tooth. Treatment of this type of lesion should involve reducing the stress that caused it in the first place, such as parafunctional habits or excessivetooth occlusion. Because enamel is stronger than dentin, dentin is frequently lost in response to force transmitted through the enamel to the DEJ.
Developmental disturbances
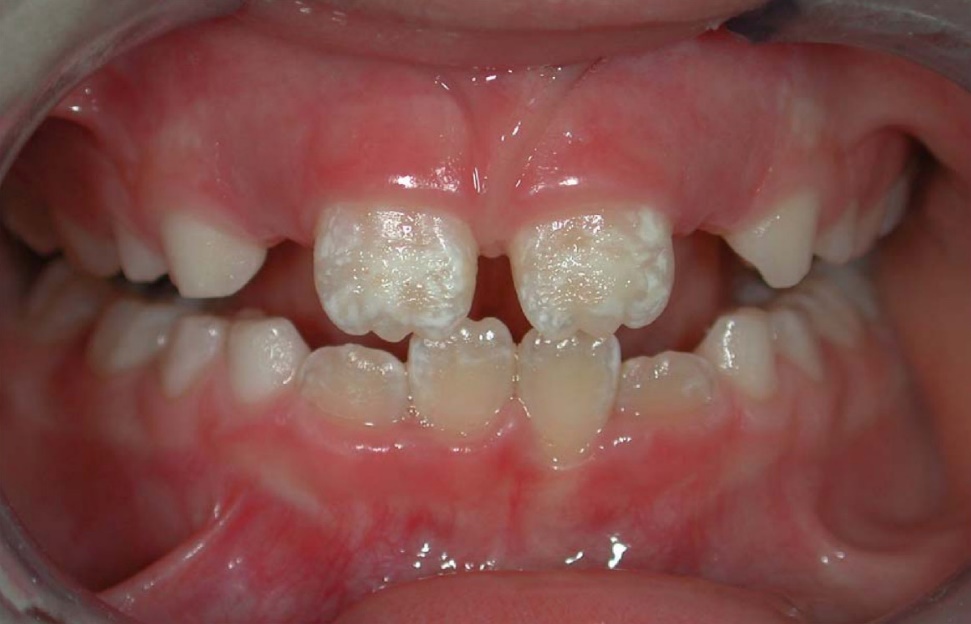
"Symmetrical enamel hypoplasia of grade I on permanent incisors in a CD patient
"
by Maurizio Procaccini et al, Head & Face Medicine
is licensed under CC BY 2.0Enamel hypoplasia
Reduced enamel formation during embryonic development is called enamel hypoplasia. This occurs to primary and/or succedaneous teeth. Hypoplasia in an embryo is often triggered by health changes in the mother, such as a gastrointestinal disorder or a syphilis infection during pregnancy. If ameloblasts cannot acquire enough minerals during the time they are active, upon tooth eruption the surface enamel may contain white lesions, or exhibit pits and grooves. The enamel is more brittle and susceptible to erosion and abfraction. Furthermore, increased sensitivity to temperature and increased incidence of dental caries and periodontal disease are expected. Because amelogenesis is complete long before birth, there is currently no way to significantly assist in enamel production after tooth eruption occurs. Therefore, preventative treatment becomes much more important, and full-coverage crowns might be required.

"Amelogenesis imperfecta
"
by 신연아 is licensed under CC BY 3.0Amelogenesis imperfecta
Another cause of enamel hypoplasia is the genetic condition amelogenesis imperfecta. There are a number of different genes that, when mutated, cause this disease. For instance, mutations in genes for amelogenins and enamelins leads to forms of amelogenesis imperfecta by reducing the ability of scaffolds to rapidly nucleate calcium hydroxyapatite crystal formation. This results in enamel that is hypo-mineralized. Additionally, gene mutations to the matrix metalloproteinases necessary for proper enamel development can result in amelogenesis imperfecta. Without removal of ameloginins and enamelins by the matrix metalloproteinase enzymes, ameloblasts cannot create the high mineral content of healthy, mature enamel. Because amelogenesis imperfecta is caused by different mutations to a number of different genes, the symptoms occur on a spectrum, from mild to severe.

"Own work"
, By Wicketcity is licensed under CC BY-SA 4.0 / croppedEnamel pearl
The IEE within HERS does not normally differentiate← into ameloblasts because of the lack of morphogens from stellate reticulum. However, a small region of the IEE might mistakenly differentiate into ameloblasts along the root and produce a small amount of enamel over cementum, known as an enamel pearl. There may be no harm in having pearls, other than they may be confused with a calculus during a scaling procedure. If the wrong technique is used, the instrument tip may break off when it encounters the much harder enamel pearl. However, if an emalep pearl forms near or in a fucrcation, there will be a void in the attachment apparatus at that site. This results most often in a periodontal pocket which can result in tooth loss, depending on the extent of the void. Enamel pearls can be identified by radiography, because enamel is more radiopaque than cementum. Enamel pearls generally occur on the furcations of the multi-rooted molars.
< Chapter 8 * navigation * Chapter 10 >
Chapter review questions
The developmental history of a differentiated cell traced back to the embryonic cell from which it arises.
Mesenchyme tissue that contains neural crest cell derivatives.
he most exterior of the three primary germ layers formed in the gastrula, it gives rise to the epithelial tissue covering the body and the CNS.
Extra-Cellular Matrix: Materials in the body, but outside of cells. A network of macromolecules, such as fibers, enzymes, and glycoproteins, that provide structural and biochemical support to surrounding cells.
Cells present only during the embryonic period that deposit tooth enamel.
A cell of neural crest origin that is part of the outer surface of the dental pulp, and whose biological function is the formation of dentin.
Shared equally by two parties.
A substance whose non-uniform distribution governs the pattern of tissue development and pattern formation.
A protein that controls the rate of transcription of DNA to mRNA, by binding to a specific DNA sequence.
The process by which animals and plants grow and change.
The mucous membrane lining the inside of the mouth. It is a stratified squamous epithelium, named the oral epithelium, and an underlying areolar connective tissue named the lamina propria.
Undifferentiated or partially differentiated cells that can differentiate into various cell types, and proliferate to produce more of the same stem cell.
When one cell begins to look different from another. This process involves limiting cell fate by altering gene transcription to become more specialized.
A medical procedure in which ground up bone tissue or synthetic bone tissue is placed in an injured site to act as a scaffold, promoting the formation of new bone tissue by the patient's own cells.
Procedures attempting to regenerate (as opposed to replace) lost periodontal structures.
A compound that has an effect on a living organism, tissue or cell, such as by mimicking a morphogen or growth factor.
Ca5(PO4)3(OH), the inorganic ECM material composed of calcium, phosphate and hydroxide ions that makes up the bulk of bone tissue.
The shape (or form) of.
A histologic landmark identified on an ameloblast.
A layer of dark granules that lie parallel to the outer surface of root dentin.
Arm-like extension of an odontoblast found within dentinal tubules.
The formation of enamel.
The stage of early tooth development where the dental organ differentiates into 4 distinct cell types 9IEE, OEE, stellate reticulum and stratum intermedium), followed by differentiation of odontoblasts and ameloblasts.
Inward folding of an epithelium caused by interstitial growth.
An epithelium composed of one layer of square cells
Inner Enamel Epithelium: a layer of columnar cells located next to the dental papilla, these pre-ameloblast cells will differentiate into Ameloblasts which are responsible for secretion of enamel during tooth development.
Outer Enamel Epithelium: a layer of cuboidal cells located on the periphery of the enamel organ in a developing tooth.
A stratified layer of cells situated between the IEE and the stellate reticulum.
A group of star-shaped cells located in the center of the enamel organ of a developing tooth, they synthesize glycosaminoglycans.
The ectodermal cells that lie above the dental papillae in a cap stage tooth bud.
A subset of mesenchymal stem cells derived from neural crest cells rather than mesoderm, or mesoderm-derived mesenchymal stem cells induced by neural crest morphogens to adopt a more neural fate.
A condensation of ecto-mesenchymal cells seen in histologic sections of a developing tooth.
A thin sheet of extracellular matrix between epithelial tissues and the underlying connective tissue of the lamina propria or dermis.
The top (or apex) side of a cell or tissue, usually facing the lumen.
The side of a cell or tissue oriented away from the lumen or external surface.
An intermediate cell type, differentiate from IEE cells, capable of inducing the differentiation of odontoblasts, which in turn induce pre-ameloblasts to differentiate into ameloblasts,.
Signaling molecules first identified for their role in carcinogenesis, then for their function in embryonic development, including body axis patterning, cell fate specification, cell proliferation and cell migration.
A polarity axis that organizes cells in the plane (side-to-side) of the tissue.
The process of cell division, where one cell divides into two identical clones (daughter cells).
Half of a desmosome, specialized for cell-to-ECM adhesion.
Bone Morphogenetic Proteins are a group of signaling molecules, initially discovered for their ability to induce bone formation, now known to play crucial roles in all organ systems.
The embryonic process in which one group of cells directs the development of another group of cells.
Newly formed dentin before calcification and maturation.
A trans-membrane protein which allows cells to bind to the ECM protein fibronectin, it is involved in cell adhesion, growth, migration, and differentiation.
The main structural protein in the ECM of connective tissues
Fibroblast Growth Factors are a family of morphogens involved in a wide variety of processes, including important roles in development and tissue regeneration, especially connective tissues.
Copying DNA into a functional product, such as a RNA and/or protein. Controlled by the activity of transcription factors binding to gene promoter regions to recruit RNA polymerase.
A sequence of nucleotides in DNA that encodes the synthesis of a gene product, either RNA or protein.
A group of 235-300 related genes that code for a transcription factors, which control the activity of other genes involved in development, including directing the formation of limbs and organs along the anterior-posterior axis.
A cell-surface, trans-membrane or cytoplasmic protein that binds to (receives) a signaling molecule and transmit the signal further.
A hard connective tissue which is mostly ECM, including collagen fibers and a calcium-phosphate crystal.
The initial un-mineralized form of enamel secreted by ameloblasts.
Proteins found in enamel matrix which regulate the initiation and growth of hydroxyapatite crystals during the mineralization of enamel.
Along with amelogenins, a second protein found in enamel, enamelins are necessary for the adhesion of ameloblasts to the surface of the enamel, and they bind to hydroxyapatite to promotes crystallite elongation.
Extracellular matrix material involved in the repair of injured and missing tissues, allows stem cells to migrate into the injured area. It is usually replaced during regeneration.
The basic connective tissue cell type capable of secreting ECM components, including fibers and ground substance proteins.
An embryonic tissue composed of undifferentiated mesenchymal stem cells and mucous ground substance.
The middle of the three embryonic layers that develop during gastrulaton, mesoderm becomes most of the body's muscle and connective tissues.
Methyl groups added to DNA which change the activity of a gene without changing the sequence, typically repressing gene transcription.
Highly basic proteins found in nuclei that compact DNA into a denser form, unavailable for gene transcription.
The process of converting DNA into an mRNA copy
Incremental growth lines seen in enamel.
A particularly pronounced incremental line in enamel or dentin, created on the day of birth.
Incremental growth lines that appear on the surface of tooth enamel as a series of linear grooves.
The basic unit of tooth enamel, 4 μm wide, composed of a tightly packed and organized mass of hydroxyapatite crystals, hexagonal in shape.
The type of enamel found between enamel rods.
The type of enamel found within an enamel rod
When cut enamel is viewed under reflected light, curves in the pattern of enamel rods can be seen; these curves strengthen the enamel and prevent cracks from propagating through the tooth.
Dentin-Enamel Junction
Enzymes that degrade all kinds of extracellular matrix proteins, or process a number of bioactive molecules. They play a major role in cell proliferation, migration, differentiation, angiogenesis, and apoptosis.
Gel-like substances in the ECM, composed of water held in place by large molecules (proteins, glycoproteins and glycosaminoglycans).
A process in tooth development in which the teeth enter the mouth and become visible.
Reorganization or renovation of existing tissues, either physiological or pathological. The process can either change the characteristics of a tissue such as in blood vessel remodeling, or result in the dynamic equilibrium of a tissue such as in bone remodeling.
A condition that causes changes in the appearance of tooth enamel. It may result when children regularly consume fluoride during the teeth-forming years, age 8 and younger.
The use of an acids to prepare enamel for the application of an adhesive, which roughens the surface and removes the smear layer, increasing retention of resin sealant.
Fingerlike projections of the apical side of the dermis, increasing the surface area between the epidermis and dermis, strengthening their connection and increasing exchange of oxygen, nutrients, and waste.
Fingerlike projections of the epidermis, the downward-pointing counterparts to the dermal papillae.
The wearing away of tooth enamel by excess acids.
The symptom of having a dry mouth due to reduced saliva production.
Damage to a tooth that can happen when decay-causing bacteria make acids that de-mineralize tooth tissues.
Materials used in surgical implants, not harmful to living tissue and unlikely to be rejected by the host immune system.
The loss of tooth structure at the CEJ, often wedge-shaped or V-shaped, and is unrelated to cavities, bacteria, or infection.
A defect of the enamel that occurs during tooth development, it results in thin enamel making teeth vulnerable to decay.
A rare congenital disorder which presents with abnormal formation of enamel, unrelated to any environmental conditions.
Hertwig's Epithelial Root Sheath: A proliferation of epithelial cells located at the cervical loop of the enamel organ in a developing tooth which initiates the formation of root dentin.
A condition of teeth where enamel is found where enamel is not supposed to be, such as on a root surface.
The tissues that attach the tooth to the alveolar process: cementum, periodontal ligament, and alveolar bone.