5.2 Chemical and Biochemical Sedimentary Rocks
Clastic sedimentary rocks are dominated by components that have been transported as solid clasts (clay, silt, sand, etc.). In contrast, chemical and biochemical sedimentary rocks are dominated by components that have been transported as ions in solution (e.g., Na+, Ca2+, HCO3–, etc.). There is some overlap between the two because almost all clastic sedimentary rocks contain cement formed from dissolved ions, and many chemical sedimentary rocks include some clasts. The difference between chemical and biochemical sedimentary rocks is that in biochemical sedimentary rocks, organisms play a role in turning the ions into sediment. This means the presence and nature of biochemical sedimentary rocks are linked to the life requirements of the organisms that comprise them. In chemical sedimentary rocks, the process is inorganic, often resulting from a body of water evaporating and concentrating the ions. It is possible for one type of sedimentary rock to form from both chemical (inorganic) and biochemical (organically mediated) processes.
Chemical and biochemical sedimentary rocks are classified based on the minerals they contain, and are frequently dominated by a single mineral. It is true that some clastic sedimentary rocks, such as quartz arenite, can also be dominated by a single mineral, but the reasons for this are different. A clastic sedimentary rock can contain whatever minerals were present in the parent rock. The minerals the clastic rock ends up containing will depend on how much “processing” the sediments undergo by physical and chemical weathering, and transport, before the sediment was cemented. On the other hand, chemical sedimentary rocks are limited largely to those minerals that are highly soluble in water. Because mineral content is a defining characteristic of chemical and biochemical sedimentary rocks, we will use it to organize our discussion of these rocks.
Carbonate Rocks
Carbonate rocks are those in which the dominant mineral contains the carbonate anion (CO32-). The main carbonate minerals are calcite and aragonite. Both minerals have the formula CaCO3 but they have different crystal structures. A less common carbonate mineral that is still important for forming carbonate rocks is dolomite, which has the formula CaMg(CO3)2. It is similar to calcite and aragonite, except that some of the calcium is replaced with magnesium. Dolomite is more common as a replacement mineral, which has replaced calcite in carbonate rocks.
Limestone
Limestone is comprised of calcite and aragonite. It can occur as a chemical sedimentary rock, forming inorganically due to precipitation, but most limestone is biochemical in origin. In fact, limestone is by far the most common biochemical sedimentary rock.
Almost all limestone forms in marine (i.e., oceans or salty seas) environments, and most of that forms on the shallow continental shelves, especially in tropical regions with coral reefs. Today continental shelves are relatively narrow zones along the margins of continents, but for large parts of geologic history sea-level was much higher, and large parts of the interiors of continents were flooded.
Reefs are highly productive ecosystems populated by a wide range of organisms, many of which use calcium and bicarbonate ions from seawater to make carbonate minerals (especially calcite) for their shells and other structures. These include corals as well as green and red algae, urchins, sponges, molluscs, and crustaceans. Some of micro-organisms use CaCO3 to build tiny tests (shells) which accumulate on the ocean floor when these organisms die. Erosion can break all of these carbonate materials apart, scattering fragments throughout surrounding region (Figure 5.9).
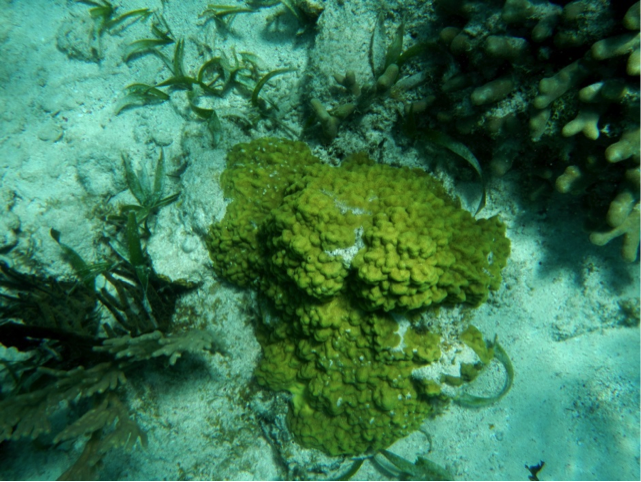
Figure 5.10 shows a cross-section through a typical reef environment in a tropical region (normally between 40° N and 40° S). Reefs tend to form near the edges of steep drop-offs because the reef organisms thrive on nutrient-rich upwelling currents. As the reef builds up, it is eroded by waves and currents to produce carbonate sediments that are transported into the steep offshore fore-reef area and the shallower inshore back-reef area. Reef-derived sediments are dominated by reef-type carbonate fragments of all sizes, including mud.
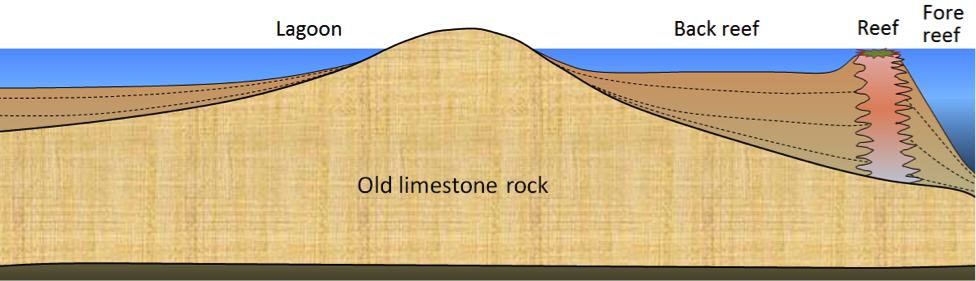
In many such areas, carbonate-rich sediments also accumulate in quiet lagoons, where mud and mollusc-shell fragments predominate (Figure 5.11, left) or in offshore areas with strong currents, where either foraminifera tests accumulate (Figure 5.11, middle) or calcite crystallizes inorganically to form ooids – spheres of calcite that form in shallow tropical ocean water with strong currents (Figure 5.11, right).
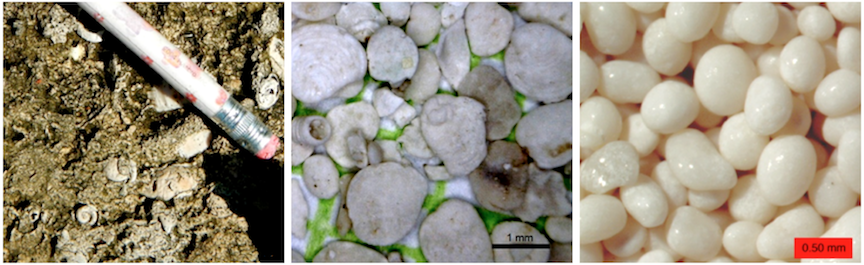
Limestone also accumulates in deeper water, from the steady settling out of the carbonate shells of tiny organisms that lived near the ocean surface. Processes on the ocean floor cause the water in the deepest parts of the ocean to become more acidic. This puts a lower limit on how deep in the ocean calcite and aragonite can accumulate, because they dissolve under acidic conditions.
Tufa and Travertine
Calcite can form chemical sedimentary rocks on land in a number of environments. Tufa forms at springs. The tufa towers in Figure 5.12 formed where spring water discharged into lake water.
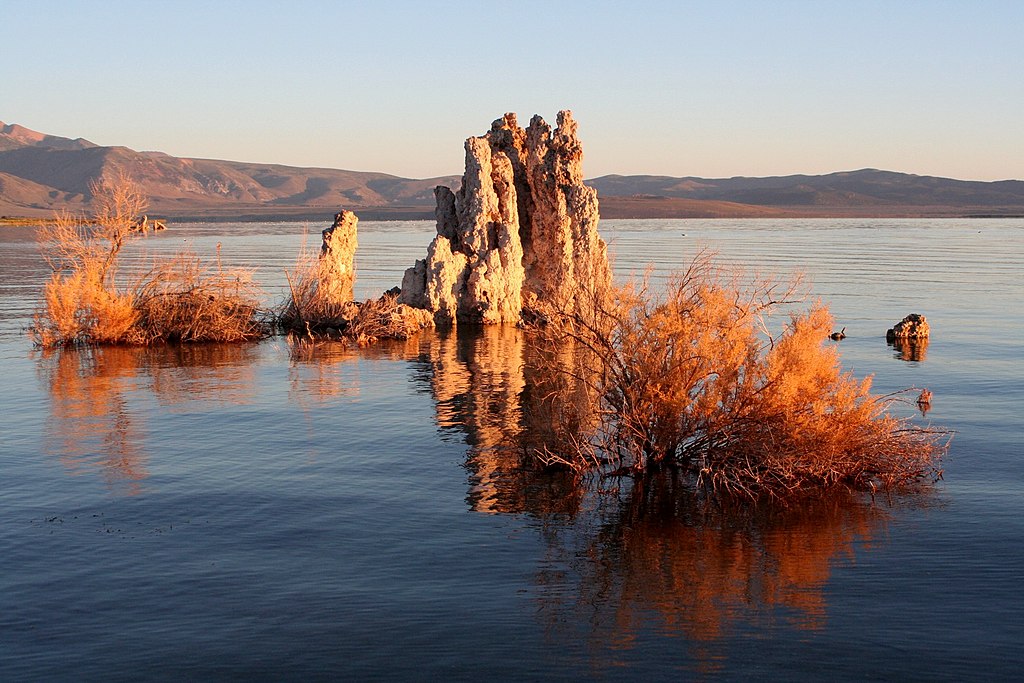
Travertine (which is less porous) forms at hot springs. Similar material precipitates within limestone caves to form speleothems (mineral deposits in caves, Figure 5.13) such as stalactites and stalagmites.
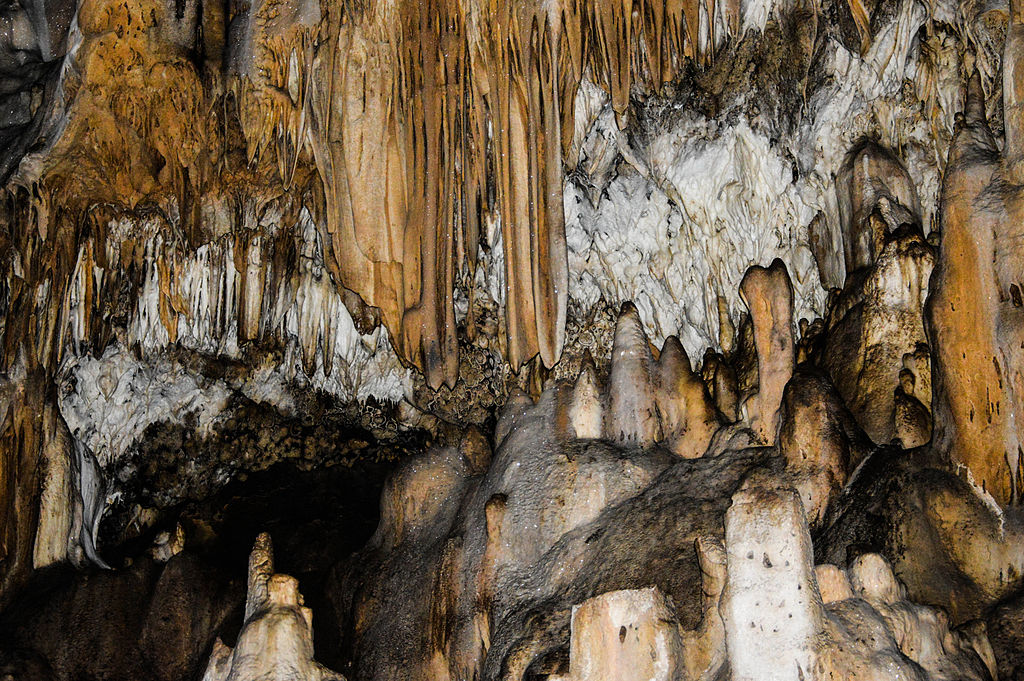
Dolostone
Dolostone (also referred to as dolomite) is the carbonate rock made of the mineral dolomite (CaMg(CO3)2). Dolostone is quite common (there’s a whole Italian mountain range named after it), which is surprising because marine organisms do not precipitate dolomite. Dolomite forms through dolomitization, a process thought to involve chemical reactions between magnesium-rich water percolating through rocks, and sediments containing calcite.
Calcite and dolomite can be distinguished from one another by applying a drop of weak acid to the rock; calcite will react with weak acid, whereas dolomite will not. Also, when dolomite weathers, it tends to turn buff (tan) in color, whereas calcite tends toward grey and white.
Chert
Chert is made of silica (SiO2). It has the same chemical formula as quartz, but is cryptocrystalline, meaning that the quartz crystals comprising chert are so small that it is difficult to see them even under a microscope. Chert can be a chemical sedimentary rock, often forming as beds within limestone (Figure 5.14), or as irregular lenses or blobs (nodules). It can also be biochemical. Some tiny marine organisms (e.g., diatoms and radiolaria) make their tests from silica. When they die their tiny shells (or tests) settle slowly to the bottom of the lake or ocean, where they accumulate and are transformed into chert.
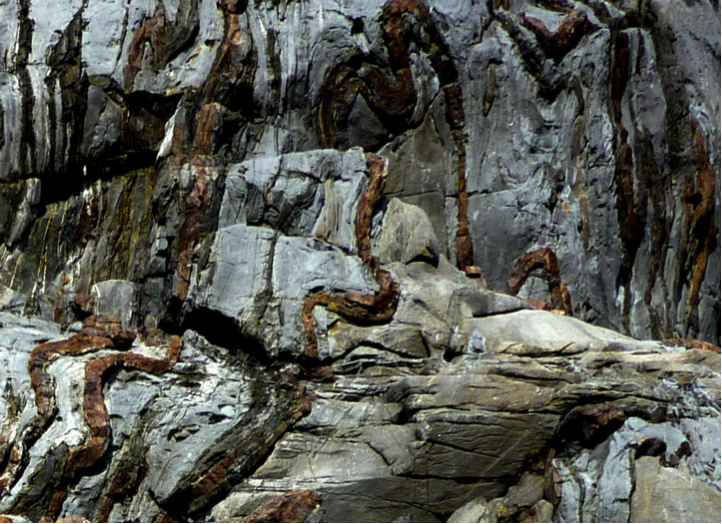
Banded Iron Formations (BIFs)
Some ancient chert beds — most dating to between 1800 and 2400 Ma — are also part of a rock known as a banded iron formation (BIF). It is a deep sea-floor deposit of iron oxide that is a common ore of iron. These rocks consist of alternating layers of dark iron oxide minerals (magnetite and hematite) and chert stained red by hematite (Figure 5.15).
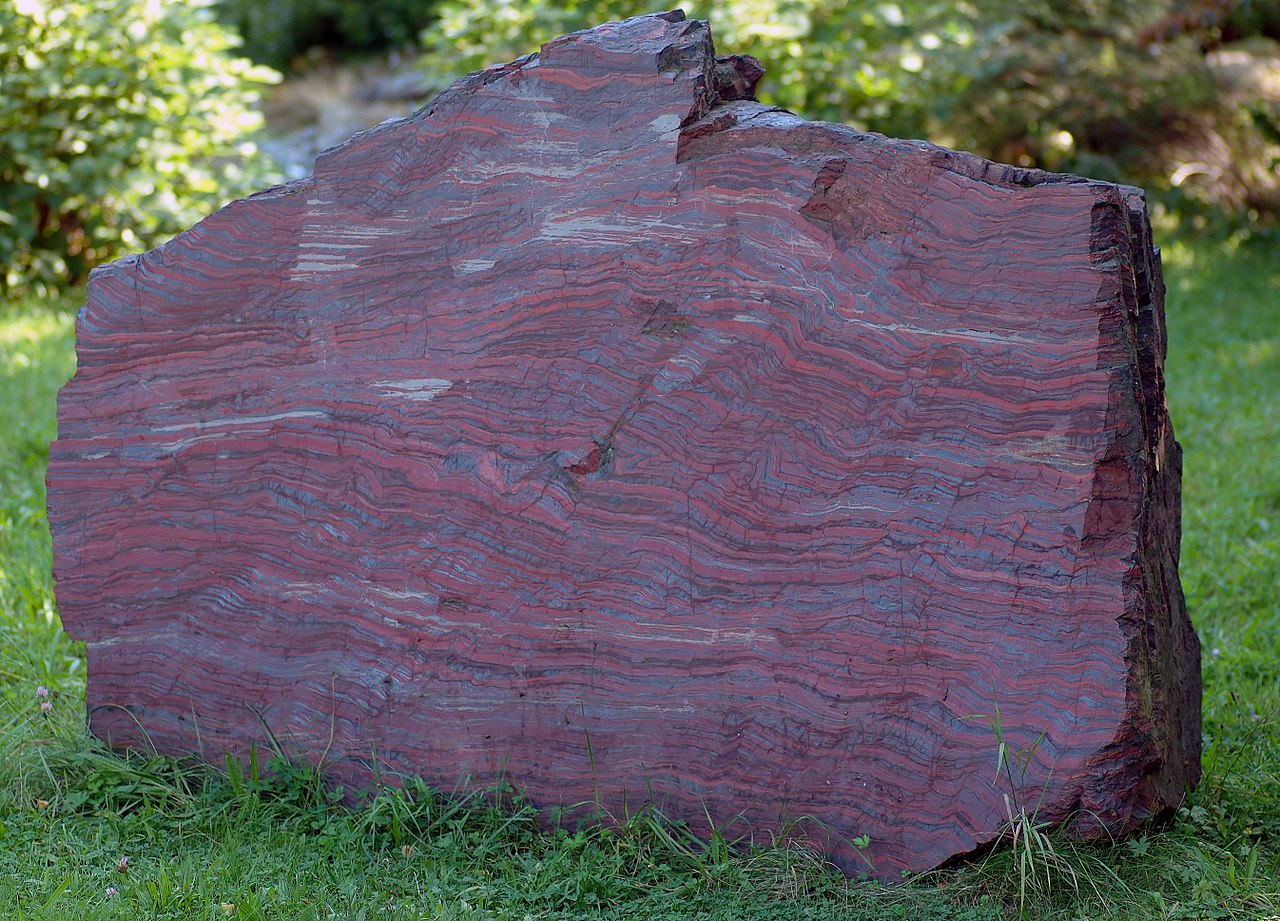
Evaporites
In arid regions, lakes and inland seas typically have no stream outlet, and the water that flows into them is removed only by evaporation. Under these conditions, the water becomes increasingly concentrated with dissolved salts, and eventually some of these salts may reach saturation levels and start to crystallize (Figure 5.16).
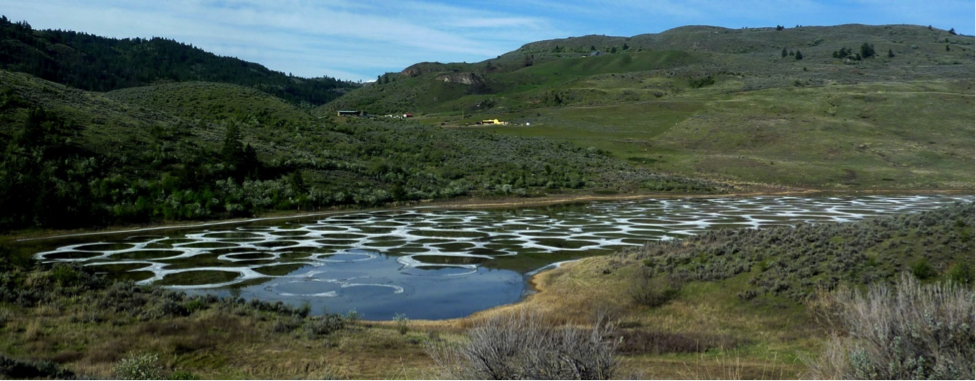
Although all evaporite deposits are unique because of differences in the chemistry of the water, in most cases minor amounts of carbonates start to precipitate when the solution is reduced to about 50% of its original volume. Gypsum (CaSO4·H2O) precipitates at about 20% of the original volume, and halite (NaCl) precipitates at 10%. Other important evaporite minerals include sylvite (KCl) and borax (Na2B4O7·10H2O). Sylvite is mined as potash at numerous locations across Saskatchewan from evaporites that formed during the Devonian (~385 Ma) when an inland sea occupied much of the region.
Exercise 5.2: Making Evaporite
This is an easy experiment that you can do at home. Pour about 50 mL (just less than 1/4 cup) of very hot water into a cup and add 2 teaspoons (10 mL) of salt. Stir until all or almost all of the salt has dissolved, then pour the salty water (leaving any undissolved salt behind) into a shallow wide dish or a small plate. Leave it to evaporate for a few days and observe the result. It may look a little like the Figure 5.17. These crystals are up to ~3 mm in diameter.
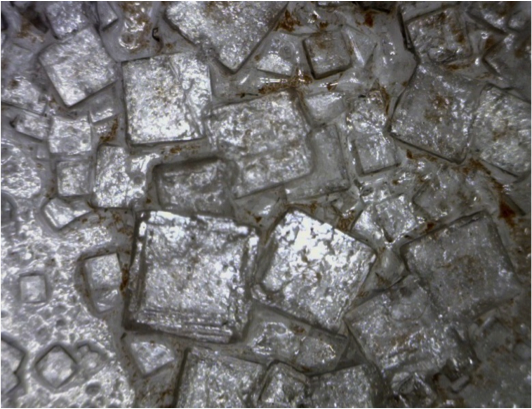
Video: Geoscience Videos – Chemical Sedimentary Rocks
Video: Geoscience Videos – Biochemical Sedimentary Rocks
Licenses and Attributions
“Physical Geology, First University of Saskatchewan Edition” by Karla Panchuk is licensed under CC BY-NC-SA 4.0 Adaptation: Renumbering