6.3 Chirality in Chemical, Environmental and Biological Systems
Chiral molecules, as we learned in the introduction to this chapter, have an interesting optical property. Physics teaches us that light waves can be described as oscillating electric and magnetic fields. In ordinary light, the oscillation is randomly oriented in an infinite number of planes. When ordinary light is passed through a polarizer, all planes of oscillation are filtered out except one, resulting in plane-polarized light.
This is the kind of polarizing filter that is used in anti-glare glasses and ski goggles.
A beam of plane-polarized light, when passed through a sample of a chiral compound, interacts with the compound in such a way that the angle of oscillation will rotate. This property is called optical activity.
If a compound rotates plane polarized light in the clockwise (+) direction, it is said to be dextrorotatory, while if it rotates light in the counterclockwise (-) direction it is levorotatory. Under standardized laboratory conditions, the specific rotation of a pure chiral compound can be measured and used as a physical property characteristic of that specific substance. This is the specific rotation for that substance:
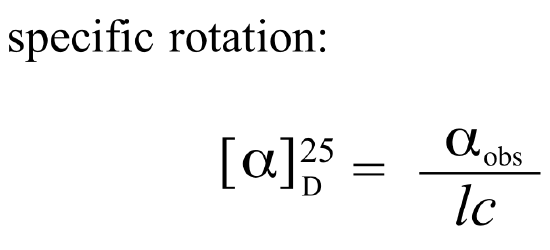
. . . where alpha(obs) is the observed rotation, l is the length of the path light must travel through the sample, and c is the concentration of the sample in grams per 100 mL.
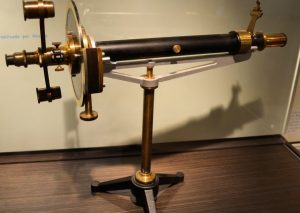
The two enantiomers of a compound will always rotate plane-polarized light with an equal but opposite magnitude. (S)-ibuprofen, for example, has a specific rotation of +54.5o (dextrorotatory) in methanol, while (R)-ibuprofen has a specific rotation of -54.5o. There is no relationship between chiral compound’s R/S designation and the direction of its specific rotation because the R/S system is not experiment-based. For example, the S enantiomer of ibuprofen is dextrorotatory, but the S enantiomer of glyceraldehyde is levorotatory.
A 50:50 mixture of two enantiomers (called a racemic mixture) will have no observable optical activity, because the equal amounts of two optical activities cancel each other out in the experimental situation.
In a structural drawing, a ‘squiggly’ bond from a chiral center indicates a mixture of both R and S configurations.
Chiral molecules are often labeled according to whether they are dextrorotatory or levorotatory as well as by their R/S designation. For example, the pure enantiomers of ibuprofen are labeled (S)-(+)-ibuprofen and (R)-(-)-ibuprofen, while (±)-ibuprofen refers to the racemic mixture, which is the form in which the drug is sold to consumers.
All of the twenty natural amino acids except glycine have a chiral center at their alpha-carbon, which is the carbon that is next-door to the carbonyl carbon. Virtually all of the amino acids found in nature, both in the form of free amino acids or incorporated into peptides and proteins, have what is referred to in the biochemical literature as the ‘L‘ configuration at this carbon:
It is unfortunate but true that biochemical literature often uses this alternative naming system, rather than sharing the R/S designations used in organic chemistry.
Chirality is everywhere in biology: in the proteins built from these chiral amino acids, in carbohydrates, in DNA, and in other organic molecules such as the signaling molecules such as neurotransmitters. Enzymes are themselves chiral, and so are able to distinguish between stereoisomers, catalyzing chemistry in some while not catalyzing chemistry in others.
While the small differences between enantiomers can be less important in a test tube, the differences matter in biological systems. This presents a great challenge to the pharmaceutical industry, which is often faced with the need to produce drugs that are specific stereoisomers because they are to be used in biological systems. Synthesizing these molecules exclusively, or separating them out from a mixture of stereoisomers can be enormously difficult. Chemical reactions occurring in test tubes and flasks without chiral reagents will produce a mixture of stereochemistry in the products. These substances are similar enough to the desired isomer that they can be very difficult to separate out.
The chemical reactions that occur within biological systems, on the other hand, are catalyzed by enzymes and produce specific stereoisomers. This is one reason the pharmaceutical industry has increased their use of fermentation and other biological production systems: they can circumvent the difficulty of separating stereoisomers if they can get a biologically-engineered microbe to produce a drug for them.
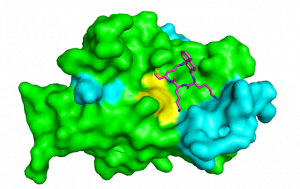