3.1 Intermolecular Forces
Until now we have been focusing on understanding the covalent bonds that hold individual molecules together. We turn next to consider the subject of non-covalent interactions between molecules, or between different functional groups within a single molecule. The forces resulting in these interactions are called intermolecular forces. Along with ion-ion and ion-dipole forces they make up a set we might call noncovalent interactions.
Understanding these intermolecular interactions will allow us to explain differences in physical properties -such as boiling points, melting points, and solubility – between different organic compounds.
As you work through this section remember that these interactions are different than the covalent bonds that hold atoms together within a molecule. These interactions do not come from electron sharing, and they are individually much, much weaker than the covalent bonds which hold a molecule together. It is their aggregate effect that is important. Similar to how the individual hook and loop interactions of a piece of Velcro are weak, while the overall stickiness of a large piece of Velcro is strong, individual weak interactions between molecules in a sample can have an enormous effect on the characteristic of the substance in bulk.
Dipoles
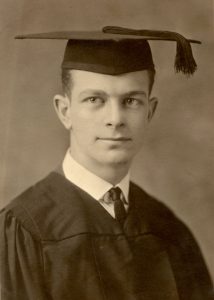
To understand the nature of intermolecular forces, we first must revisit covalent
bonds and delve into the subject of dipoles. Many of the covalent bonds that we have seen – between two carbons, for example, or between a carbon and a hydrogen –involve the approximately equal sharing of electrons between the two atoms in the bond. In these examples, the two atoms have approximately the same electronegativity. Linus Pauling, a famous chemist, Oregonian, and a key developer of these ideas, described electronegativity as “the power of an atom in a molecule to attract electrons to itself.”
Quite often in organic chemistry we deal with covalent bonds between two atoms with different electronegativities, and in these cases the sharing of electrons is not equal. The more electronegative atom pulls the bonding electrons closer to its nucleus. In the carbon-oxygen bond of an alcohol, for example, the two electrons are held more closely to the oxygen than they are to the carbon, because oxygen is significantly more electronegative than carbon. The same is true for the oxygen-hydrogen bond, as hydrogen is much less electronegative than oxygen.
The result of this unequal sharing is what we call a bond dipole, which exists in a polar covalent bond. The unequal sharing of the bonding electrons produces negative and positive ends, or poles, where electron density is lower (the positive pole) and higher (the negative pole). The resulting charges are called ‘partial positive’ and ‘partial negative.’ ‘Partial’ is used because these charges are not as great as the numeric charges we assign to elctrons (-1) or protons (+1).
When dipoles are shown on chemical structures, the difference in electron density can be expressed using either of two types of notation. The Greek letter delta to denote the poles, with a plus (electron deficient) or minus (electron rich) sign is one way. The other uses ‘dipole arrows’, with a positive sign on the tail, to indicate the negative (higher electron density) direction of the dipole.
The degree of polarity in a covalent bond depends on the difference in electronegativity between the two bonded atoms. Electronegativity is a periodic trend: it increases going from left to right across a row of the periodic table of the elements, and also increases as we move up a column. Therefore, oxygen is more electronegative than nitrogen, which is in turn more electronegative than carbon. Oxygen is also more electronegative than sulfur. Fluorine, in the top right corner of the periodic table, is the most electronegative of the elements. Hydrogen is slightly less electronegative than carbon.
Periodic trends in electronegativity
Most molecules contain both polar and nonpolar covalent bonds. Depending on the location of polar bonds and bonding geometry, molecules may posses a net polarity, called a molecular dipole moment. Water has a dipole moment that results from the combined dipoles of its two oxygen-hydrogen bonds. Fluoromethane also has a dipole moment.
Tetrafluoromethane, however, has four polar bonds that pull equally in to the four corners of a tetahedron, meaning that although there are four individual bond dipoles there is no overall molecular dipole moment. Carbon dioxide also lacks a molecular dipole moment.
Dipole moments can be calculated, and are described with a value and the unit “D” for Debye. (Debye is pronounced so it would rhyme with “the spy.”)
Exercise 3.1.1
Types of intermolecular forces
Ion-ion, dipole-dipole and ion-dipole forces
The strongest type of non-covalent interaction is between two ionic groups of opposite charge (an ion-ion or charge-charge interaction). For example, table salt is composed of sodium cations and chloride anions, held in a crystal lattice by ion-ion attractive interactions. One of the most common examples in biological organic chemistry is the interaction between a magnesium cation (Mg+2) and an anionic carboxylate or phosphate group. The figure below shows a polyatomic anion named 2-phosphoglycerate interacting with two Mg+2 ions as it does in biological organisms, in the active site of an enzyme.
Polar molecules – those with a molecular dipole moment, such as acetone – can align themselves in such a way as to allow their respective positive and negative poles to interact with each other. This is called a dipole-dipole interaction.
When a charged species (an ion) interacts favorably with a polar molecule or functional group, the result is called an ion-dipole interaction. A common example of ion-dipole interaction in biological organic chemistry is that between a metal cation, most often Mg+2 or Zn+2, and the partially negative oxygen of a carbonyl.
London dispersion forces
Nonpolar molecules such as hydrocarbons also are subject to weaker but still significant attractive intermolecular attractive forces. London dispersion forces, also called van der Waal’s forces or nonpolar interactions result from the constantly shifting electron density in any molecule. Even a nonpolar molecule will, at any given moment, have a weak, short-lived dipole. This transient dipole will induce a neighboring nonpolar molecule to develop a corresponding transient dipole of its own, with the end result that a transient dipole-dipole interaction is formed. These forces are very weak, but in the absence of other intermolecular forces they do matter.
Hydrogen Bonding forces
Hydrogen bonds result from the interaction between a hydrogen bonded to a very electronegative heteroatom – specifically a nitrogen, oxygen, or fluorine – and lone-pair electrons on a nitrogen, oxygen, or fluorine a neighboring molecule or functional group. Because a hydrogen atom is just a single proton and a single electron, when it loses electron density in a polar bond it essentially becomes an approximation of a ‘naked’ proton, capable of forming an unusually strong noncovalent interaction with a lone pair on a neighboring electronegative atom.
Hydrogen bonds are usually depicted with dotted lines in chemical structures. A group that provides a proton to a hydrogen bond is said to be acting as a hydrogen bond donor. A group that provides an oxygen or nitrogen lone pair is said to be acting as a hydrogen bond acceptor. Many common organic functional groups can participate in the formation of hydrogen bonds, either as donors, acceptors, or both. Water and alcohols, for example, can be both hydrogen bond donors and acceptors.
Hydrogen bonding has a huge importance in biology. Copying of DNA in the cell, for example, is based on very specific hydrogen bonding arrangements between DNA bases on complimentary strands: adenine pairs with thymine, while guanine pairs with cytidine:
Exercise 3.1.2
Exercise 3.1.3
Draw figures that show the hydrogen bonds described below. If you are unable to draw them, describe them with words.
a) A hydrogen bond between methanol (donor) and water (acceptor).
b) A hydrogen bond between methanol (acceptor) and water (donor).
Relative Strength of Intermolecular Forces
In general, hydrogen bonding forces are stronger than dipole-dipole forces, but are also much weaker than covalent bonds. The strength of hydrogen bonds has enormous implications in biology.
Dipole-dipole interactions are somewhat weaker than Hydrogen bonding interactions.
Least powerful are the London dispersion forces that hold nonpolar substances together. These interactions occur all the time, but their effect is overshadowed by the more powerful intermolecular forces when a substance is capable of dipole-dipole interactions or Hydrogen bonding.
Exercise 3.1.4