3.2 Solubility
An understanding of the various types of noncovalent intermolecular forces allows us to explain many observable physical properties of organic compounds on a molecular level. One physical property that has links to intermolecular forces is solubility. Whether some organic substance will dissolve in a liquid solvent, and to what extent it will do so, is linked to the structures of the molecules making up this solute and the solvent.
Solubility
A lot of organic chemistry takes place in the solution phase. In the organic laboratory, reactions are often run in nonpolar or slightly polar solvents such as toluene (methylbenzene), dichloromethane, or diethyl ether. In recent years, much effort has been made to adapt reaction conditions to allow for the use of more environmentally friendly solvents such as water or ethanol, which are polar and capable of hydrogen bonding. So laboratory chemistry tends to occur in these environments.
In biochemistry the solvent is of course water, but the microenvironment inside an enzyme’s active site – where the actual chemistry is going on – can range from very polar to very non-polar, depending on which amino acid residues on the enzyme surround the reactants.
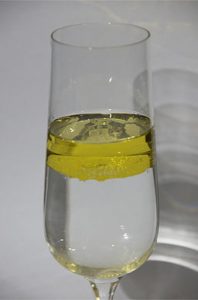
You have probably observed at some point in your life that oil does not mix with water, either in a puddle underneath a car with a leaky oil pan, or in a vinaigrette dressing bottle in the kitchen. The underlying reason for this insolubility (or immiscibility when we talk about liquids) is intermolecular forces that exist (or don’t) between molecules within the solute, the solvent, and between the solute and solvent.
When considering the solubility of an organic compound in a given solvent, the most important question to ask ourselves is: How strong are the noncovalent attractive interactions between the compound and the solvent molecules? If the solvent is polar, like water, then a larger dipole moment, indicating greater molecular polarity, will tend to increase the solubility of a substance in it. If the solvent is non-polar, like the hydrocarbon hexane, then the exact opposite is true.
Imagine that you have a flask filled with water, and a selection of substances that you will test to see how well they dissolve in it. The first substance is table salt, or sodium chloride. This ionic compound dissolves readily in water. Why? Because water, as a very polar molecule, is able to form many ion-dipole interactions with both the sodium cation and the chloride anion, the energy from which is more than enough to make up for energy required to break up the ion-ion interactions in the salt crystal.
The end result, then, is that in place of sodium chloride crystals, we have individual sodium cations and chloride anions surrounded by water molecules – the salt is now in solution. Charged species as a rule dissolve readily in water: in other words, they are very hydrophilic (water-loving).
Biphenyl, like sodium chloride, is a colorless crystalline substance.
Biphenyl does not dissolve at all in water. Why is this? It is a very non-polar molecule, with only carbon-carbon and carbon-hydrogen bonds. It has some intermolecular forces bonding it to itself through nonpolar London dispersion forces, but it has no significant attractive interactions with very polar solvent molecules like water. Meanwhile the water molecules themselves are highly connected to one another through hydrogen bonding forces. Thus, the water tends to continue to engage in hydrogen bonding interactions with other molecules of its own kind, and very little is gained in terms of new biphenyl-water interactions. Water is a terrible solvent for nonpolar hydrocarbon molecules: they are very hydrophobic (water-fearing).
Next, you try a series of increasingly large alcohol compounds, starting with methanol (1 carbon) and ending with octanol (8 carbons).
You find that the smaller alcohols – methanol, ethanol, and propanol – dissolve easily in water, at any water/alcohol ratio that you try. This is because the water is able to form hydrogen bonds with the hydroxyl group in these molecules, and the increased stability in the system due to formation of these water-alcohol hydrogen bonds is more than enough to make up for the lost stability from undoing the alcohol-alcohol (and water-water) hydrogen bonds. When you try butanol, however, you begin to notice that, as you add more and more to the water, it starts to form a layer on top of the water. Butanol is only sparingly soluble in water.
The longer-chain alcohols – pentanol, hexanol, heptanol, and octanol – are increasingly insoluble in water. What is happening here? Clearly, the same favorable water-alcohol hydrogen bonds are still possible with these larger alcohols. The difference, of course, is that the larger alcohols have larger nonpolar, hydrophobic regions in addition to their hydrophilic hydroxyl group. At about four or five carbons, the influence of the hydrophobic part of the molecule begins to overcome that of the hydrophilic part, and water solubility is lost.
Now, try dissolving glucose in the water – even though it has six carbons just like hexanol, it also has five hydrophilic hydroxyl (-OH) groups that can engage in hydrogen bonding interactions, in addition to a sixth oxygen that is capable of being a hydrogen bond acceptor.
We have tipped the scales to the hydrophilic side, and we find that glucose is quite soluble in water.
We saw that ethanol was very water-soluble (if it were not, drinking beer or vodka would be rather inconvenient!) How about dimethyl ether, which is a constitutional isomer of ethanol but with an ether rather than an alcohol functional group? We find that diethyl ether is much less soluble in water. Is it capable of forming hydrogen bonds with water? Yes, in fact, it is –the ether oxygen can act as a hydrogen-bond acceptor. The difference between the ether group and the alcohol group, however, is that the alcohol group is both a hydrogen bond donor and acceptor.
The result is that the alcohol is able to form more energetically favorable interactions with the solvent compared to the ether, and the alcohol is therefore much more soluble.
‘Like dissolves like’ is a general rule for solubility frequently taught in chemistry classes. This phrase consolidates the patterns described above, and while it loses some of the explanation and is really general, it is helpful.

‘Like’ items are those that are more polar, or capable of hydrogen bonding or interacting with ions. Polar solvents will dissolve polar substances well, and also ionic ones. Nonpolar substances, in contrast, will not: but they will do a good job of dissolving things that are nonpolar.
Nonpolar solvents are less familiar to non-chemists, but in daily life they do sometimes help when it is necessary to dissolve something nonpolar. For instance, essential oils are oil solutions of fragrance molecules because the fragrance compounds are nonpolar and will not dissolve in water. Cleaning solvents also often are at least somewhat nonpolar, and help to dissolve and therefore remove nonpolar greasy contaminants from tools, bikes, and other places around the house. In the environment, oils tend to float on water and thus can cover wide areas rather than remain confined to a local spill. Small volumes of spilled hazardous materials that are nonpolar can contaminate vast areas.
Summary of factors contributing to water solubility
Evaluating a chemical structure to predict its solubility characteristics can be challenging. But consideration of these factors can often lead to predictions that match real observed behavior of substances:
A: How many carbons? All else being equal, more carbons means more of a non-polar/hydrophobic character, and thus lower solubility in water.
B: How many, and what kind of hydrophilic groups? The more, the greater the water solubility. In order of importance:
- Anything with a charged group (eg. ammonium, carboxylate, phosphate) is almost certainly water soluble, unless has a vary large nonpolar group, in which case it will most likely be soluble in the form of micelles, like a soap or detergent.
- Any functional group that can donate a hydrogen bond to water (e.g. alcohols, amines) will significantly contribute to water solubility.
- Any functional group that can only accept a hydrogen bond from water (eg. ketones, aldehydes, ethers) will have a somewhat smaller but still significant effect on water solubility.
- Other groups that contribute to polarity (eg. alkyl halides, thiols, sulfides) will make a small contribution to water solubility.
Watch for heteroatoms in molecules, which often are built into functional groups that contribute to molecular polarity, and thus water-solubility.
Exercise 3.2.1
Exercise 3.2.2
Exercise 3.2.3
Exercise 3.2.4