8 Chemical Bonds
Atoms can form several types of chemical bonds. These bonds are interactions between two atoms that hold the atoms together. It is important to understand the various types of bonds because they help determine how different molecules function within an organism. There are four types of bonds or interactions: covalent, ionic, hydrogen bonds, and van der Waals interactions.
Covalent Bonds
Another type of strong chemical bond between two or more atoms is a covalent bond. These bonds form when an electron is shared between two elements. Covalent bonds are the strongest (*see note below) and most common form of chemical bond in living organisms.
The hydrogen and oxygen atoms that combine to form water molecules are bound together by strong covalent bonds. The electron from the hydrogen atom shares its time between the hydrogen atom and the oxygen atom. In order for the oxygen atom to be stable, two electrons from two hydrogen atoms are needed, hence the subscript “2” in H2O. H2O means that there are 2 hydrogen atoms bonded to 1 oxygen atom (the 1 is implied below the O in the chemical formula). This sharing makes both the hydrogen and oxygen atoms more chemically stable.
There are two types of covalent bonds: polar and nonpolar (Figure 3). Nonpolar covalent bonds form between two atoms that share the electrons equally so there is no overall charge on the molecule. For example, an oxygen atom can bond with another oxygen atom. This association is nonpolar because the electrons will be equally shared between each oxygen atom. Another example of a nonpolar covalent bond is found in the methane (CH4) molecule. The carbon atom shares electrons with four hydrogen atoms. The carbon and hydrogen atoms all share the electrons equally, creating four nonpolar covalent bonds (Figure 3).
In a polar covalent bond, the electrons shared by the atoms spend more time closer to one atom than to the other. Because of the unequal distribution of electrons between the atoms, a slightly positive (δ+) or slightly negative (δ–) charge develops. The covalent bonds between hydrogen and oxygen atoms in water are polar covalent bonds. The shared electrons spend more time near the oxygen than they spend near the hydrogen. This means that the oxygen has a small negative charge while the hydrogens have a small positive charge.
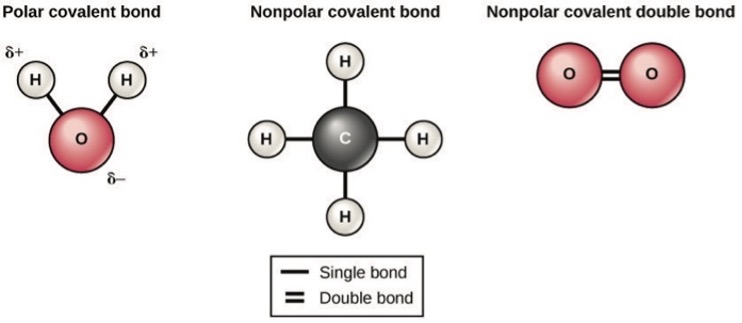
*BUT WAIT! In chemistry, I learned that Ionic bonds are stronger than covalent bonds! What’s up with that?
Turns out that chemists and biologists measure bond strength in different ways. Chemists measure the absolute strength of a bond (kind of like the theoretical strength). Ionic bonds are very strong when measured this way. Biologists are more interested in how the bond behaves in a biological system, which is usually aqueous (water-based). In water, ionic bonds come apart much more readily than covalent bonds, so biologists would say that they are weaker.
So what’s the right answer? Depends on whether you’re in a chemistry or a biology class! If you look in a biology textbook, it will almost always tell you that covalent bonds are stronger. If you look in a chemistry textbook, you’ll see something different. This is a great example of how the same information can lead to different answers depending on the perspective that you’re viewing it from.
So what answer should you give for this class? Because this is a biology class, you should say that covalent bonds are stronger than ionic bonds because they act stronger in aqueous solutions.
Ionic Bonds
Atoms normally have an equal number of protons (positive charge) and electrons (negative charge). This means that atoms are normally uncharged because the number of positively charged particles equals the number of negatively charged particles. When an atom does not contain equal numbers of protons and electrons, it will have a net charge. An atom with a net charge is called an ion. Positive ions are formed by losing electrons. Negative ions are formed by gaining electrons. Atoms can lose and donate electrons in order to become more stable.
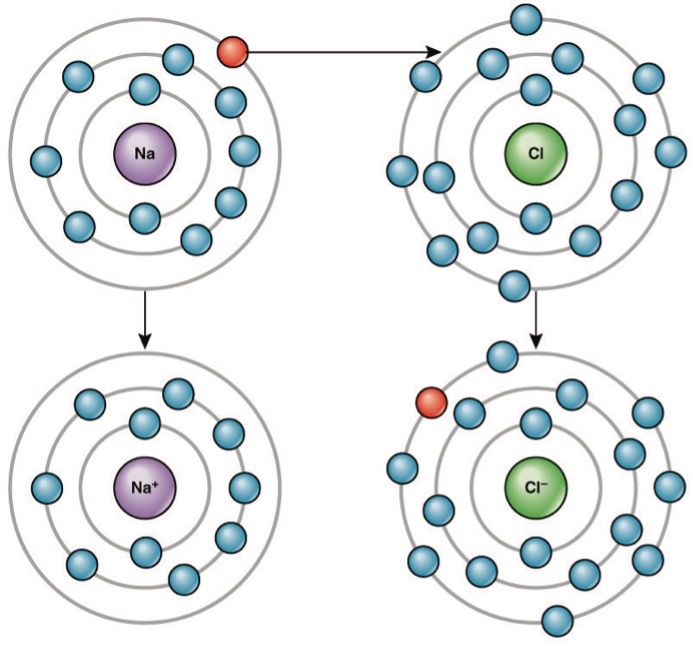
When an element donates an electron from its outer shell, as in the sodium atom example above, a positive ion is formed (Figure 2). The element accepting the electron is now negatively charged. Because positive and negative charges attract, these ions stay together and form an ionic bond, or a bond between ions. The elements bond together with the electron from one element staying predominantly with the other element. When Na and Cl combine to produce NaCl, an electron from a sodium atom goes to stay with the other seven electrons in the chlorine atom, forming a positively charged sodium ion and a negatively charged chlorine ion. The sodium and chloride ions attract each other.
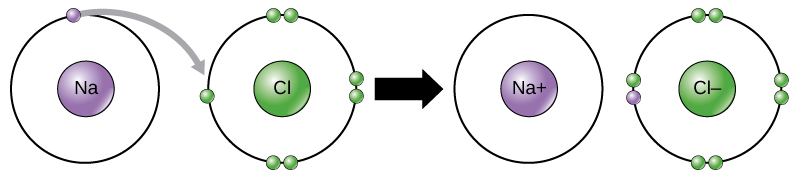
https://www.youtube.com/watch?v=OTgpN62ou24
Hydrogen Bonds
Ionic and covalent bonds are strong bonds that require considerable energy to break. However, not all bonds between elements are ionic or covalent bonds. Weaker bonds can also form. These are attractions that occur between positive and negative charges that do not require much energy to break. Two weak bonds that occur frequently are hydrogen bonds and van der Waals interactions. These bonds give rise to the unique properties of water and the unique structures of DNA and proteins.
When polar covalent bonds containing a hydrogen atom form, the hydrogen atom in that bond has a slightly positive charge. This is because the shared electron is pulled more strongly toward the other element and away from the hydrogen nucleus. Because the hydrogen atom is slightly positive (δ+), it will be attracted to neighboring negative partial charges (δ–). When this happens, a weak interaction occurs between the δ+ charge of the hydrogen atom of one molecule and the δ– charge of the other molecule. This interaction is called a hydrogen bond. This type of bond is common; for example, the liquid nature of water is caused by the hydrogen bonds between water molecules (Figure 4). Hydrogen bonds give water the unique properties that sustain life. If it were not for hydrogen bonding, water would be a gas rather than a liquid at room temperature.
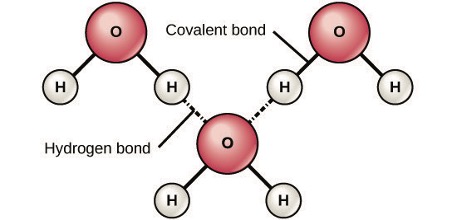
Hydrogen bonds can form between different molecules and they do not always have to include a water molecule. Hydrogen atoms in polar bonds within any molecule can form bonds with other adjacent molecules. For example, hydrogen bonds hold together two long strands of DNA to give the DNA molecule its characteristic double-stranded structure. Hydrogen bonds are also responsible for some of the three-dimensional structure of proteins.
van der Waals Interactions
Like hydrogen bonds, van der Waals interactions are weak attractions or interactions between molecules. They occur between polar, covalently bound, atoms in different molecules. Some of these weak attractions are caused by temporary partial charges formed when electrons move around a nucleus. These weak interactions between molecules are important in biological systems.
References
Unless otherwise noted, images on this page are licensed under CC-BY 4.0 by OpenStax.
OpenStax, Concepts of Biology. OpenStax CNX. March 22, 2017 https://cnx.org/contents/s8Hh0oOc@9.21:IBRqRY3C@8/The-Building-Blocks-of-Molecul