73 Structure of the Plasma Membrane
Cells closely control the exchange of substances in and out of the cell. Some substances are excluded, others are taken in, and still others are excreted – all in controlled quantities. Although the plasma membrane (cell membrane) encloses the cell’s borders, it is far from being a static barrier; it is dynamic and constantly in flux. The plasma membrane must be sufficiently flexible to allow certain cells, such as red blood cells and white blood cells, to change shape as they pass through narrow capillaries. In addition to these more obvious functions, the surface of the plasma membrane carries markers which allow cells to recognize one another. This is vital as these markers play a role in the “self” versus “non-self” distinction of the immune response.
Fluid Mosaic Model
In 1972, S. J. Singer and Garth L. Nicolson proposed a new model of the plasma membrane. This theory, compared to earlier theories, best explains both microscopic observations and the function of the plasma membrane. This theory is called the fluid mosaic model. The model has evolved somewhat over time, but still best accounts for the structure and functions of the plasma membrane as we now understand them. The fluid mosaic model describes the structure of the plasma membrane as comprised of diverse components—including phospholipids, cholesterol, proteins, and carbohydrates—that are able to flow and change position, while maintaining the basic integrity of the membrane. Both phospholipid molecules and embedded proteins are able to move laterally in the membrane. The fluidity of the plasma membrane is necessary for the activities of certain enzymes and transport molecules within the membrane.
Plasma membranes range from 5–10 nm thick. As a comparison, human red blood cells, visible via light microscopy, are approximately 8 μm thick, or approximately 1,000 times thicker than a plasma membrane.
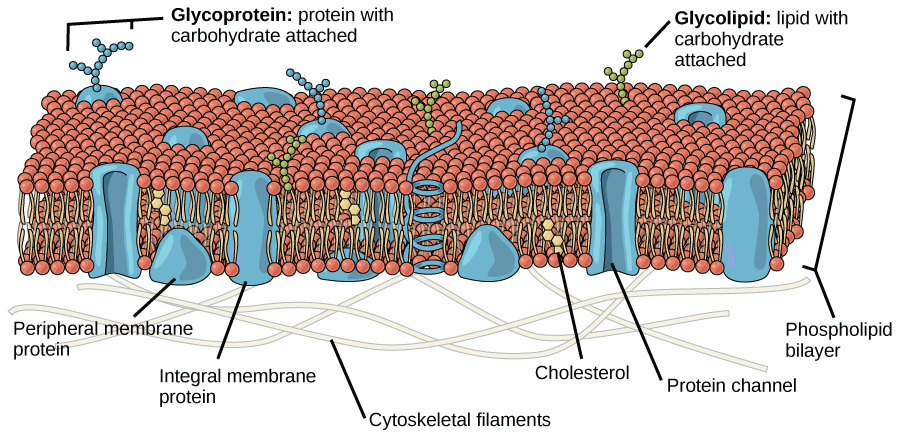
Components of the Plasma Membrane
The plasma membrane is made up primarily of a bilayer of phospholipids with embedded proteins, carbohydrates, glycolipids, and glycoproteins, and, in animal cells, cholesterol (Figure 1).
Phospholipids
The main fabric of the membrane is composed of two layers of phospholipid molecules, and the polar ends of these molecules (which look like a collection of balls in an artist’s rendition of the model) (Figure 2) are in contact with aqueous fluid both inside and outside the cell. Thus, both surfaces of the plasma membrane are hydrophilic (“water loving”). In contrast, the interior of the membrane, between its two surfaces, is a hydrophobic (“water fearing”) or nonpolar region because of the fatty acid tails. This region has no attraction for water or other polar molecules.
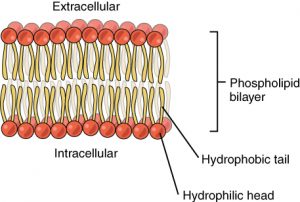
A phospholipid molecule (Figure 3) consists of a three-carbon glycerol backbone with two fatty acid molecules attached to carbons 1 and 2, and a phosphate-containing group attached to the third carbon. This arrangement gives the overall molecule an area described as its head (the phosphate-containing group), which has a polar character or negative charge, and an area called the tail (the fatty acids), which has no charge. The head can form hydrogen bonds, but the tail cannot.

Proteins
Proteins make up the second major chemical component of plasma membranes (see Figure 1). Proteins are embedded in the plasma membrane and can go all the way through the membrane or be found on one side or the other (Figure 1). Proteins may serve as enzymes, as structural attachments for the fibers of the cytoskeleton, or as part of the cell’s recognition sites. Proteins that go all the way through the membrane can serve as channels or pumps to move materials into or out of the cell. Proteins are also found on either the exterior or interior surfaces of membranes where they can be attached either to other proteins or to phospholipid molecules (Figure 1).
Five different types of proteins that are commonly associated with the cell membrane are described below.
Recognition (Identification) Proteins
Glycoproteins are proteins that have sugar molecules attached to them. Glycoproteins help cells recognize each other. The unique patterns of sugar molecules on the cell surface can be ‘read’ by corresponding glycoproteins on another cell. However, this process is different from how we read words. It’s more like fitting a key into a lock; glycoproteins will often bind together if they’re a match. This binding process communicates to the cell that it has found match. This is what helps our immune system recognize foreign invaders and then defend our body against them. The reverse is true, too. Viruses use glycoproteins to bind to and infect a host cell. Glycoprotein recognition also helps in reproduction.
Receptor Proteins
Cell surface receptors (membrane receptors, transmembrane receptors) are receptors that are embedded in the membranes of cells. A receptor is a type of recognition protein that can selectively bind a specific molecule outside the cell and this binding induces a chemical reaction within the cell. A ligand is the specific molecule that binds to and activates a receptor. The ligand molecules may be hormones, neurotransmitters, cytokines, growth factors, cell adhesion molecules, or nutrients; they react with the receptor to induce changes in the metabolism and activity of a cell.
Each receptor is structured to bind with a specific substance. The binding of a specific substance to its receptor on the plasma membrane can activate processes within the interior of the cell – such as activating enzymes involved in metabolic pathways. These metabolic pathways might be vital for providing the cell with energy, making substances for the cell, or breaking down cellular waste or toxins for disposal. Likewise, extracellular hormones and neurotransmitters bind to plasma membrane receptors which transmit a signal into the cell to intracellular molecules. Some recognition sites are used by viruses as attachment points. Although they are highly specific, pathogens like viruses may evolve to exploit receptors to gain entry to a cell by mimicking the specific substance that the receptor is meant to bind. This specificity helps to explain why human immunodeficiency virus (HIV) or any of the five types of hepatitis viruses invade only specific cells.
Movement Proteins
Channel proteins allow the movement of materials from one side of the membrane to the other, without requiring energy. The molecule being moved is taken in on one side of the channel protein, and without using energy, the molecule is released into the cell. Channels are used for facilitated diffusion which is transport especially for large polar molecules and charged ions that cannot diffuse freely across cell membranes due to the hydrophobic nature of the fatty acid tails of the phospholipids that make up the bilayers.
Carrier proteins use energy to move molecules across the membrane. The chemical to be transported must first bind at a binding site on the carrier protein. Following binding, the carrier will take in and retain the material being moved. Next, the carrier protein changes shape so that the opening in the protein now faces the other side of the plasma membrane. The material being transported is then released on that side of the membrane.
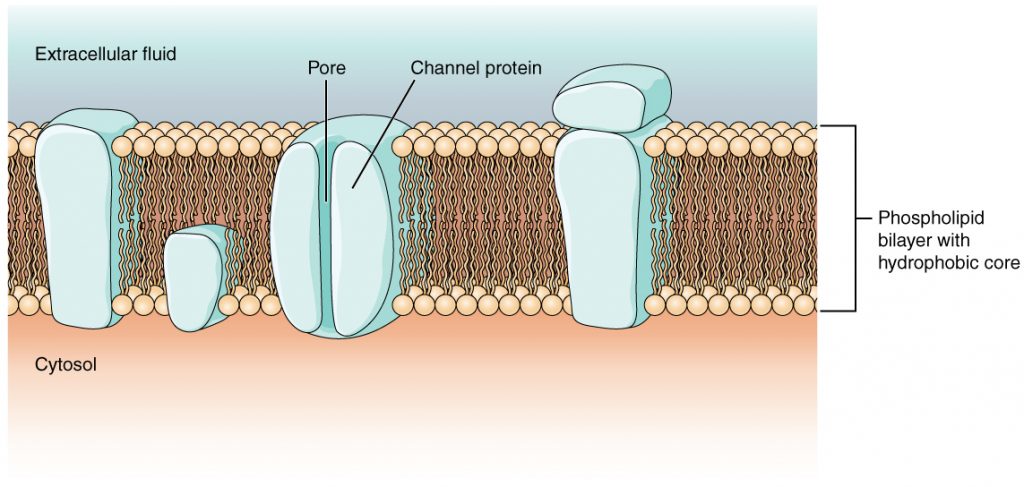
Aquaporins also called water channels is a transmembrane protein that selectively conducts water molecules in and out of the cell, while preventing the passage of ions and other solutes. Water molecules travel through the pore of the channel in single file (Figure 5). The presence of aquaporins increases membrane permeability to water. Water molecules traverse through the pore of the channel in single file. The presence of water channels increases membrane permeability to water.
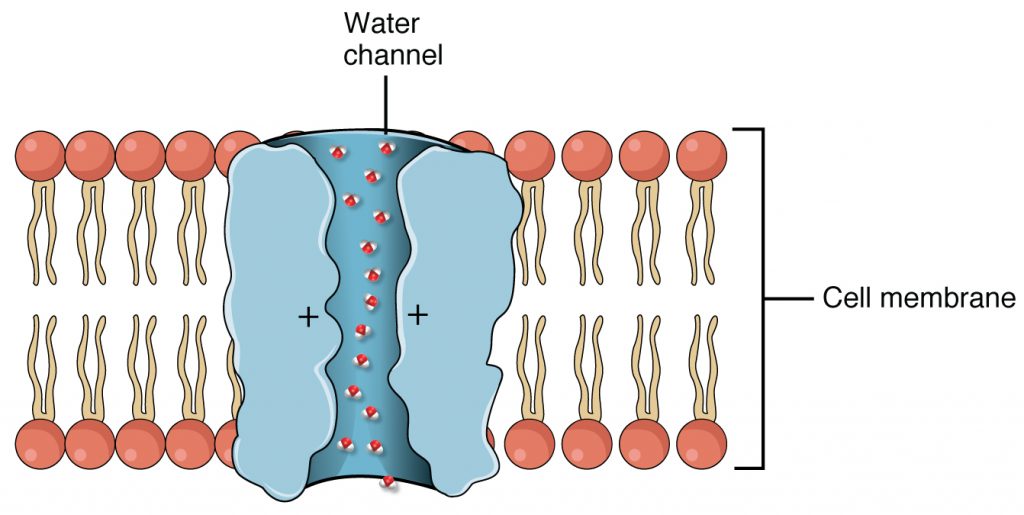
Cystic Fibrosis is caused by a defect in an integral protein in the cell membrane which acts as a channel. The CFTR protein moves ions from one side of the membrane to another. When it is not functioning correctly, this causes very thick mucus to build up in the lungs and digestive tract.
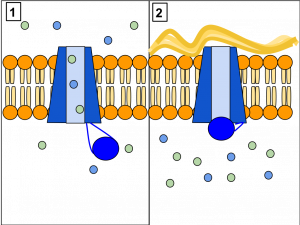
Carbohydrates
Carbohydrates are the third major component of plasma membranes. They are always found on the exterior surface of cells and are bound either to proteins (forming glycoproteins) or to lipids (forming glycolipids). These carbohydrate chains may consist of 2–60 monosaccharide units and may be either straight or branched.
Glycoproteins and glycolipids form specialized sites on the cell surface that allow cells to recognize each other. These sites have unique patterns that allow the cell to be recognized, much the way that the facial features unique to each person allow him or her to be recognized. This recognition function is very important to cells, as it allows the immune system to differentiate between body cells (called “self”) and foreign cells or tissues (called “non-self”). Similar types of glycoproteins and glycolipids are found on the surfaces of viruses and may change frequently, preventing immune cells from recognizing and attacking them.
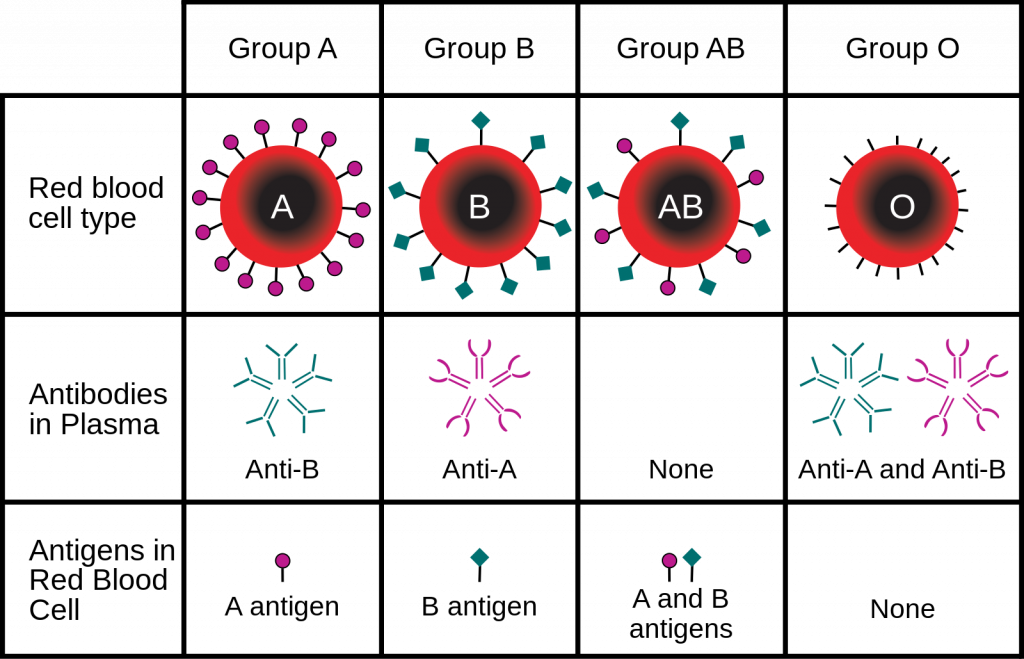
Membrane Fluidity
The mosaic characteristic of the membrane, described in the fluid mosaic model, helps to illustrate its nature. The proteins and other components that exist in the membrane can move with respect to each other, rather like boats floating on a lake. The membrane is not like a balloon, however, that can expand and contract; rather, it is fairly rigid and can burst if penetrated or if a cell takes in too much water. However, because of its mosaic nature, a very fine needle can easily penetrate a plasma membrane without causing it to burst, and the membrane will flow and self-seal when the needle is extracted.
The mosaic characteristics of the membrane explain some but not all of its fluidity. There are two other factors that help maintain this fluid characteristic. One factor is the nature of the phospholipids themselves. The structure of the fatty acid tails in each phospholipid can make the membrane more dense and rigid, or less dense and flexible. The relative fluidity of the membrane is particularly important in a cold environment. A cold environment tends to make membranes less fluid and more susceptible to rupturing. Many organisms (fish are one example) are capable of adapting to cold environments by changing the proportion of different types of fatty acids in their membranes in response to the lowering of the temperature.
Animals have an additional membrane constituent that assists in maintaining fluidity. Cholesterol, which lies alongside the phospholipids in the membrane, tends to dampen the effects of temperature on the membrane. Thus, this lipid functions as a buffer, preventing lower temperatures from inhibiting fluidity and preventing increased temperatures from increasing fluidity too much. Thus, cholesterol extends, in both directions, the range of temperature in which the membrane is appropriately fluid and consequently functional. Cholesterol also serves other functions, such as organizing clusters of proteins into lipid rafts.
References
Unless otherwise noted, images on this page are licensed under CC-BY 4.0 by OpenStax.
Text adapted from: OpenStax, Concepts of Biology. OpenStax CNX. May 18, 2016 http://cnx.org/contents/b3c1e1d2-839c-42b0-a314-e119a8aafbdd@9.10