The Light Independent Reactions (aka the Calvin Cycle)
After the energy from the sun is converted and packaged into ATP and NADPH, the cell has the fuel needed to build carbohydrate molecules. The carbohydrate molecules made will have a backbone of carbon atoms. Where does the carbon come from? The carbon atoms used to build carbohydrate molecules comes from carbon dioxide, which diffuses into the leaves through the stomata. The Calvin cycle is the term used for the reactions of photosynthesis that use the energy stored by the light-dependent reactions to form glucose and other carbohydrate molecules (Figure 1).
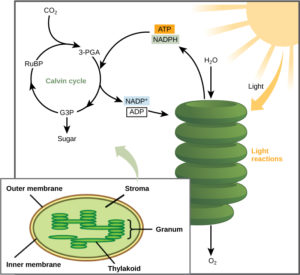
The Interworkings of the Calvin Cycle
In plants, carbon dioxide (CO2) enters the chloroplast through the stomata and diffuses into the stroma of the chloroplast—the site of the Calvin cycle reactions where sugar is synthesized. The reactions are named after the scientist who discovered them, and reference the fact that the reactions function as a cycle. Others call it the Calvin-Benson cycle to include the name of another scientist involved in its discovery.
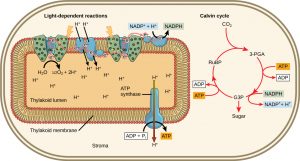
The Calvin cycle reactions (Figure 2) can be organized into three basic stages: fixation, reduction, and regeneration. In the stroma, in addition to CO2, two other molecules are present to initiate the Calvin cycle: an enzyme abbreviated RuBisCO (which stands for ribulose-1,5-bisphosphate carboxylase/oxygenase, in case you’re interested), and the molecule ribulose bisphosphate (RuBP). RuBP has five atoms of carbon and a phosphate group on each end.
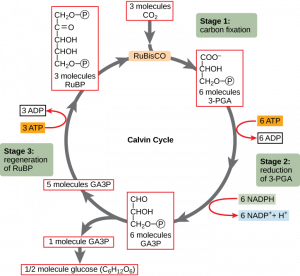
RuBisCO catalyzes a reaction between CO2 and RuBP, which forms a six-carbon compound that is immediately converted into two three-carbon compounds. This process is called carbon fixation, because CO2 is “fixed” from its inorganic form into organic molecules. You can think this as the carbon being converted from the “broken” form in CO2 (which organisms are not able to directly use) into a “fixed” form, which organisms are able to utilize. Because of this very important role in photosynthesis, RuBisCO is probably the most abundant enzyme on earth.
ATP and NADPH use their stored energy to convert the three-carbon compound, 3-PGA, into another three-carbon compound called G3P. This type of reaction is called a reduction reaction, because it involves the gain of electrons. A reduction is the gain of an electron by an atom or molecule. The molecules of ADP and NAD+, resulting from the reduction reaction, return to the light-dependent reactions to be re-energized.
One of the G3P molecules leaves the Calvin cycle to contribute to the formation of the carbohydrate molecule, which is commonly glucose (C6H12O6). Because the carbohydrate molecule has six carbon atoms, it takes six turns of the Calvin cycle to make one carbohydrate molecule (one for each carbon dioxide molecule fixed). The remaining G3P molecules regenerate RuBP, which enables the system to prepare for the carbon-fixation step. ATP is also used in the regeneration of RuBP.
In summary, it takes six turns of the Calvin cycle to fix six carbon atoms from CO2. These six turns require energy input from 12 ATP molecules and 12 NADPH molecules in the reduction step and 6 ATP molecules in the regeneration step.
Evolution Connection
Photosynthesis in desert plants has evolved adaptations that conserve water. In the harsh dry heat, every drop of water must be used to survive. Because stomata must open to allow for the uptake of CO2, water escapes from the leaf during active photosynthesis. Desert plants have evolved processes to conserve water and deal with harsh conditions. A more efficient use of CO2 allows plants to adapt to living with less water. Some plants such as cacti can prepare materials for photosynthesis during the night by a temporary carbon fixation/storage process, because opening the stomata at this time conserves water due to cooler temperatures. In addition, cacti have evolved the ability to carry out low levels of photosynthesis without opening stomata at all, an extreme mechanism to face extremely dry periods.
Section Summary
Using the energy carriers formed in the first steps of photosynthesis, the light-independent reactions, or the Calvin cycle, take in CO2 from the environment. An enzyme, RuBisCO, catalyzes a reaction with CO2 and another molecule, RuBP. After three cycles, a three-carbon molecule of G3P leaves the cycle to become part of a carbohydrate molecule. The remaining G3P molecules stay in the cycle to be regenerated into RuBP, which is then ready to react with more CO2. Photosynthesis forms an energy cycle with the process of cellular respiration. Plants need both photosynthesis and respiration for their ability to function in both the light and dark, and to be able to interconvert essential metabolites. Therefore, plants contain both chloroplasts and mitochondria.
References
Unless otherwise noted, images on this page are licensed under CC-BY 4.0 by OpenStax.
Text adapted from: OpenStax, Concepts of Biology. OpenStax CNX. May 18, 2016 http://cnx.org/contents/b3c1e1d2-839c-42b0-a314-e119a8aafbdd@9.10