68 Numerical Simulation of Skydiving Motion*
Our goal for this chapter is to understand how we created the previously shown graphs of acceleration, velocity, and position of our example skydiver, even though the net force and acceleration changes throughout. We will use a numerical simulation that ties together just about everything we have learned so far in this unit to achieve this goal. We already know that the initial velocity is zero and therefore the initial drag force is zero. With no drag force in the first moment of the jump, the diver is in free fall and the acceleration is just g in the downward direction, or -9.8 m/s/s . We can then calculate the velocity after a short time interval as:
We have made theassumption that the acceleration during this interval was constant, even though it wasn’t, but if we choose a time interval that is very small compared to the time over which the acceleration changes significantly, then our result is a good approximation. A time interval of one second will satisfy this condition in our case, so we now calculate the velocity at the end of the first one second interval:
Now that we have a velocity we can calculate the air resistance at the start of the second interval using our previously stated values for human drag coefficient, cross-sectional area, and the standard value for air density:
Now that we have a drag force due to air resistance we can use Newton's Second Law to calculate the acceleration at the start of the second interval. We have only two forces, drag and gravity and we will use our previously stated skydiver mass of 80 kg:
Now we just continue this iterative process of using acceleration and velocity values from the previous interval to calculate new velocity, drag force, and acceleration for next interval.
Using the data produced by the simulation we can graph the drag force. Showing the weight on the same graph we can see how the drag force approaches the weight.
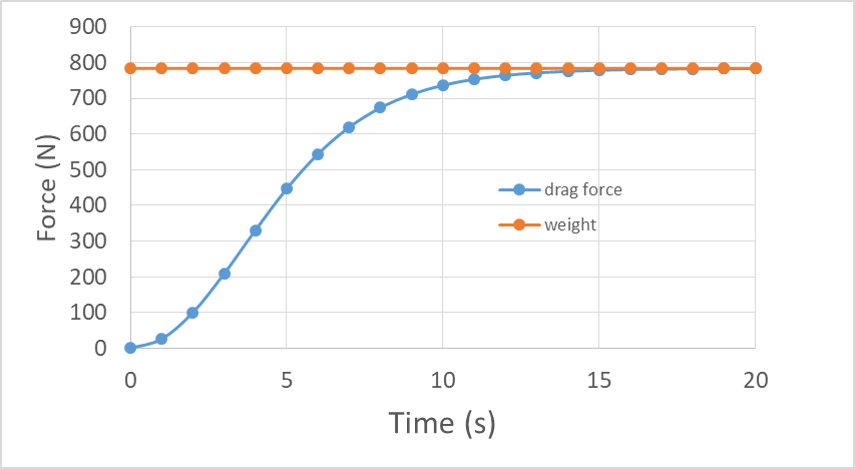
We can also use the data to create motion graphs for the skydive and see that the acceleration gradually transitions from -9.8 m/s/s to zero as drag force increases.
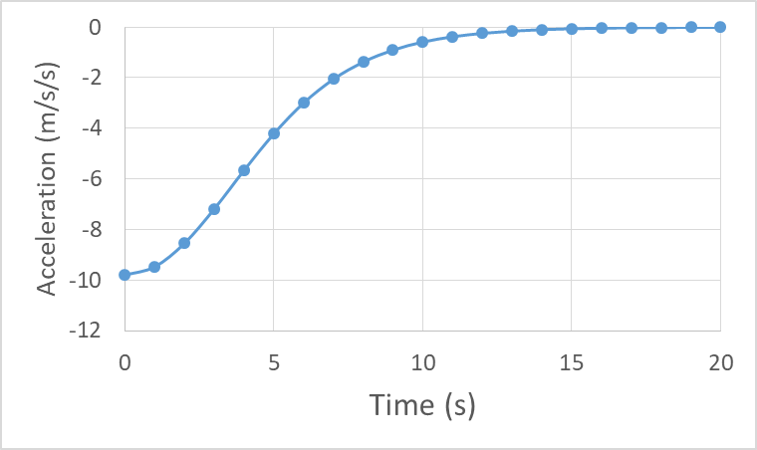
We see that velocity is always negative and the speed is always increasing, but the slope becomes less steep because the acceleration is decreasing with time:

Finally we can see that the position graph eventually becomes linear as terminal velocity is reached. (Note that we have converted our initial position of 12,000 ft to the equivalent 3660 m)
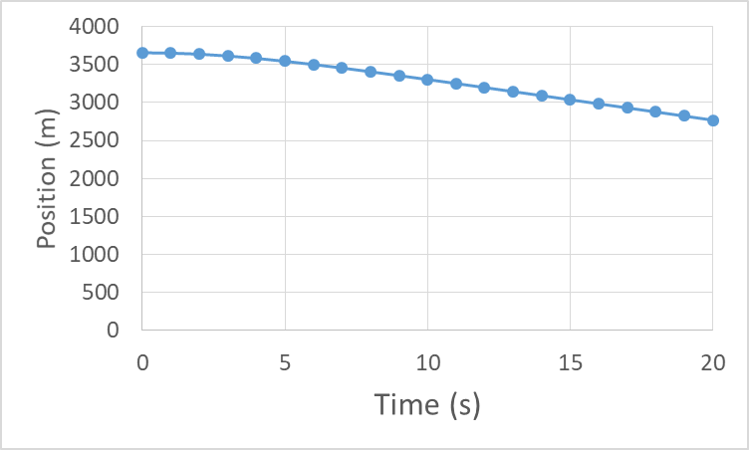
the change in velocity per unit time, the slope of a velocity vs. time graph
a quantity of speed with a defined direction, the change in speed per unit time, the slope of the position vs. time graph
location in space defined relative to a chosen origin, or location where the value of position is zero
a calculation that is run on a computer following a program that implements a mathematical model for a physical system
the value of velocity at the start of the time interval over which motion is being analyzed
a force applied by a fluid to any object moving with respect to the fluid, which acts opposite to the relative motion of the object relative to the fluid
ignoring some compilation of the in order to simplify the analysis or proceed even though information is lacking
not changing, having the same value within a specified interval of time, space, or other physical variable
a rough value obtained without making a measurement by using prior knowledge and assumptions.
a force acting opposite to the relative motion of any object moving with respect to a surrounding fluid
a number characterizing the effect of object shape and orientation on the drag force, usually determined experimentally
The cross-sectional area is the area of a two-dimensional shape that is obtained when a three-dimensional object - such as a cylinder - is sliced perpendicular to some specified axis at a point. For example, the cross-section of a cylinder - when sliced parallel to its base - is a circle
relation between the amount of a material and the space it takes up, calculated as mass divided by volume.
the acceleration experienced by an object is equal to the net force on the object divided my the object's mass
a measurement of the amount of matter in an object made by determining its resistance to changes in motion (inertial mass) or the force of gravity applied to it by another known mass from a known distance (gravitational mass). The gravitational mass and an inertial mass appear equal.
relating to or involving repetition of a mathematical or computational procedure applied to the result of a previous application
the force of gravity on on object, typically in reference to the force of gravity caused by Earth or another celestial body
distance traveled per unit time
the steepness of a line, defined as vertical change between two points (rise), divided by the horizontal change between the same two points (run)
position at the start of the time interval over which motion is being analyzed